To trap CO₂, you can use direct air capture, which filters the greenhouse gas directly from the atmosphere, or capture it at power plants through chemical absorption with amines or membrane separation. Once captured, CO₂ can be stored underground in geological formations or transformed into useful products like fuels or materials. Effective methods vary, and ongoing innovations aim to improve efficiency and lower costs—if you keep exploring, you’ll uncover even more about these exciting technologies.
Key Takeaways
- Direct Air Capture removes CO₂ directly from the atmosphere using fans and contactors, storing or converting it into products.
- Capture at power plants involves post-combustion, pre-combustion, or oxyfuel methods to isolate CO₂ from emissions.
- Underground storage injects supercritical CO₂ into geological formations like saline reservoirs or depleted oil fields for secure containment.
- Chemical absorption with amines uses solutions that react with CO₂, which is then released through heating to regenerate the absorbent.
- Membrane separation employs specialized materials to selectively allow CO₂ to pass through, offering an energy-efficient capture option.
Direct Air Capture (DAC) Technologies
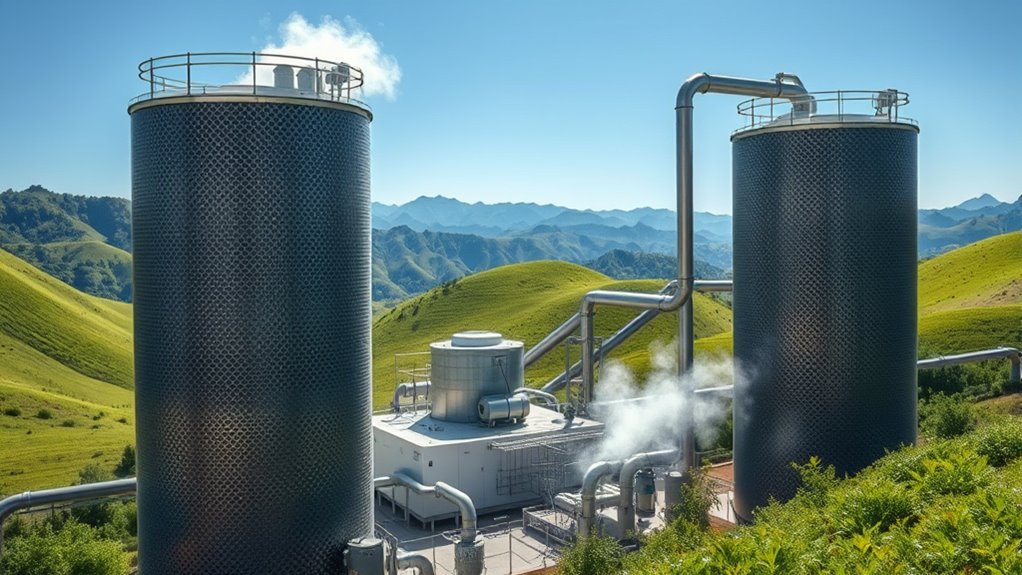
Direct Air Capture (DAC) technologies actively remove CO₂ directly from the atmosphere, unlike traditional methods that target emissions at their source. You can deploy DAC systems anywhere, giving you flexibility to locate facilities away from emission sources.
The captured CO₂ can be stored underground permanently or converted into useful products like fuels or building materials. These systems operate at low to medium temperatures, depending on the method used—solid sorbents need about 80-120°C, while liquid solutions require higher temperatures up to 900°C. Understanding regional legal resources can help facilitate permitting and compliance for DAC installations.
Large fans pull ambient air through contactors or filters that selectively trap CO₂ molecules. The captured CO₂ is then purified and prepared for storage or utilization. Current DAC technologies are rapidly evolving to improve efficiency and reduce costs, making them more viable for large-scale deployment. To keep operations sustainable, energy sources like renewables or waste heat power these facilities, minimizing their overall carbon footprint.
Capturing CO₂ at Power and Industrial Plants
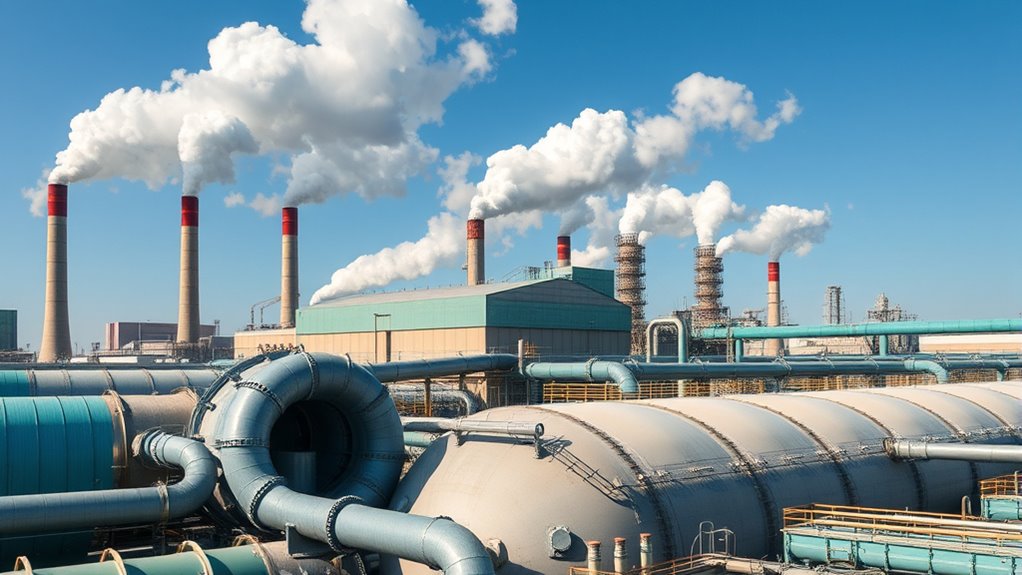
Capturing CO₂ directly from power and industrial plants plays a key role in reducing overall emissions from fossil fuel use. Post-combustion capture is common in existing power plants, where it isolates CO₂ from flue gases after burning fuel. Technologies can achieve over 90% efficiency but increase energy use, reducing overall plant performance. CO₂ must then be compressed for transport or storage. This process is proven and has been implemented at commercial scale for decades. Pre-combustion capture involves gasifying fuels to produce syngas, separating CO₂ before combustion, often in industries like hydrogen production. Oxyfuel combustion burns fuel in pure oxygen, creating a CO₂-rich flue gas that’s easier to capture, with efficiencies over 90%. These methods enable significant emission reductions but require infrastructure for compression, transport, and underground storage to make sure CO₂ doesn’t enter the atmosphere.
Chemical Absorption With Amines
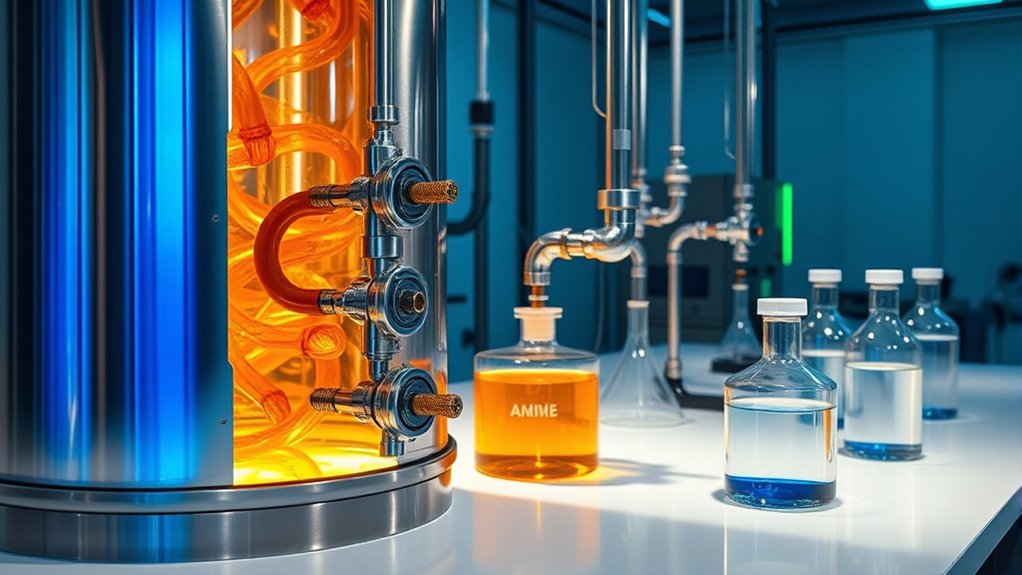
Chemical absorption with amines is a widely used method for removing CO₂ from flue gases in industrial processes. You expose the flue gas to an aqueous amine solution, like monoethanolamine (MEA), which reacts with CO₂ to form soluble carbonate salts.
This reaction is reversible, allowing you to regenerate the amine solution by heating it in a stripper column, releasing the captured CO₂. The process involves an absorption column where gases are cooled to improve efficiency, and a reboiler that supplies heat for regeneration.
While this method offers high removal efficiency and is well-understood, it demands significant energy for regeneration and can cause equipment corrosion over time. Despite these challenges, chemical absorption with amines remains a reliable and scalable solution for CO₂ capture.
Gas Separation via Membranes
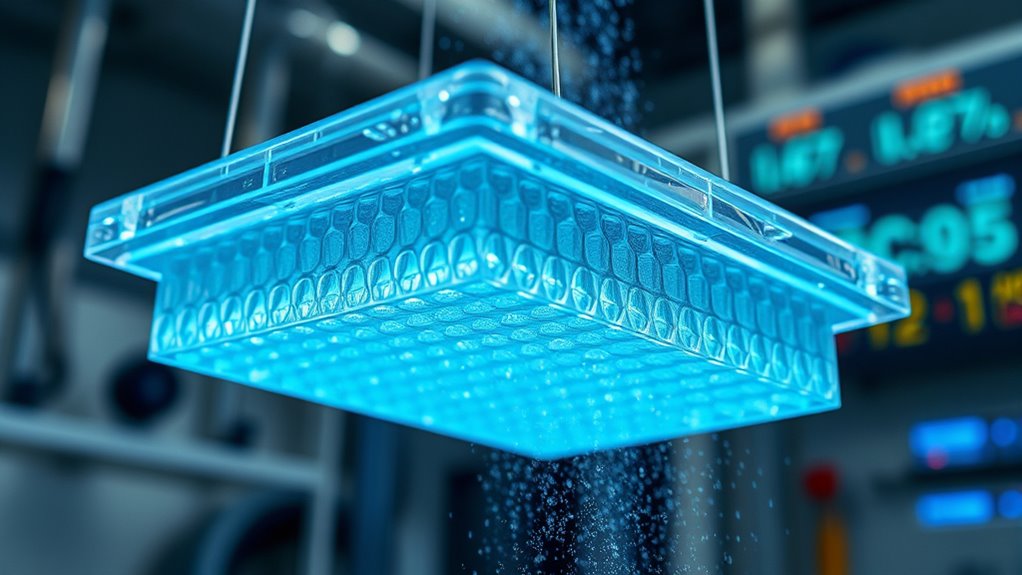
Gas separation via membranes offers an alternative approach to removing CO₂ from industrial emissions, complementing chemical absorption methods. Using permeable or semi-permeable materials, membranes selectively allow CO₂ to diffuse based on differences in diffusion rates and surface adsorption. This process is energy-efficient and simple to operate, with no moving parts, reducing maintenance needs. Membrane systems have lower capital costs and a smaller plant footprint, making them adaptable through modular designs that ease scale-up. Advances focus on improving membrane materials for better selectivity and permeability, enhancing overall efficiency. Despite challenges like pressure requirements and contamination, ongoing research aims to reduce costs and improve durability. Membrane separation is increasingly being adopted in various industries due to its energy efficiency and scalability. Additionally, ongoing innovations aim to enhance membrane selectivity and permeability, further improving performance. Collaborations between industry leaders and innovators are driving the development of more effective membrane technologies for carbon capture applications.
Storing CO₂ Underground
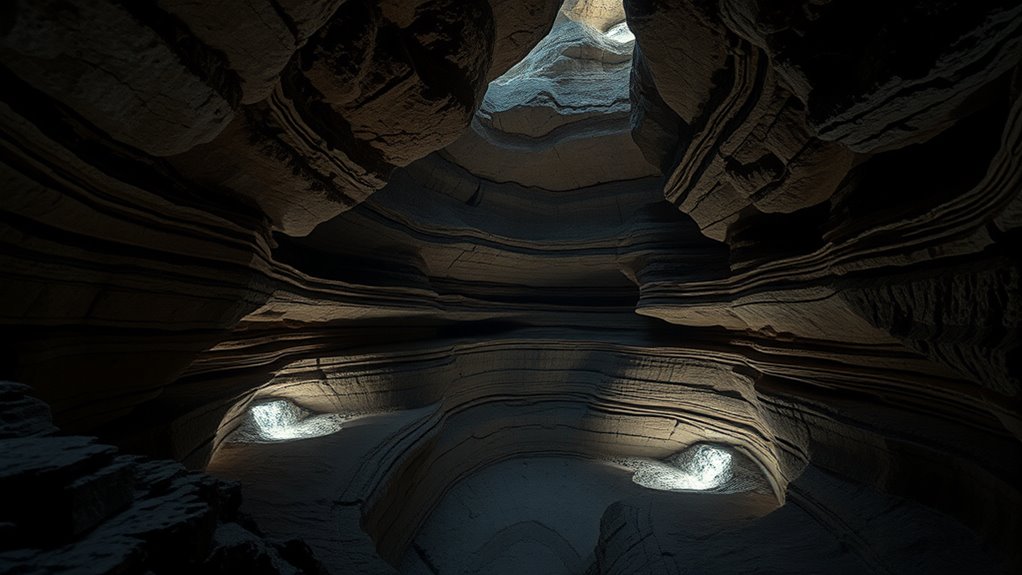
Storing CO₂ underground involves injecting it into suitable geological formations where it can be securely trapped for the long term. You’ll typically target saline formations, depleted oil and gas reservoirs, unmineable coal seams, or volcanic rocks, usually more than 3,000 feet deep. These sites are ideal because they maintain CO₂ in a supercritical state, reducing storage volume. The process begins with compressing CO₂ to become supercritical, then injecting it into porous rocks that displace existing fluids like brine. Containment relies on cap rocks that act as barriers, preventing CO₂ from migrating upward. Trapping mechanisms such as residual, solubility, and mineral trapping further secure the CO₂. Ongoing monitoring—using seismic imaging, sensors, and sampling—ensures the CO₂ stays safely contained and leaks are detected early. Implementing advanced monitoring technologies enhances the safety and effectiveness of storage sites over time.
Transforming Co₂ Into Useful Products
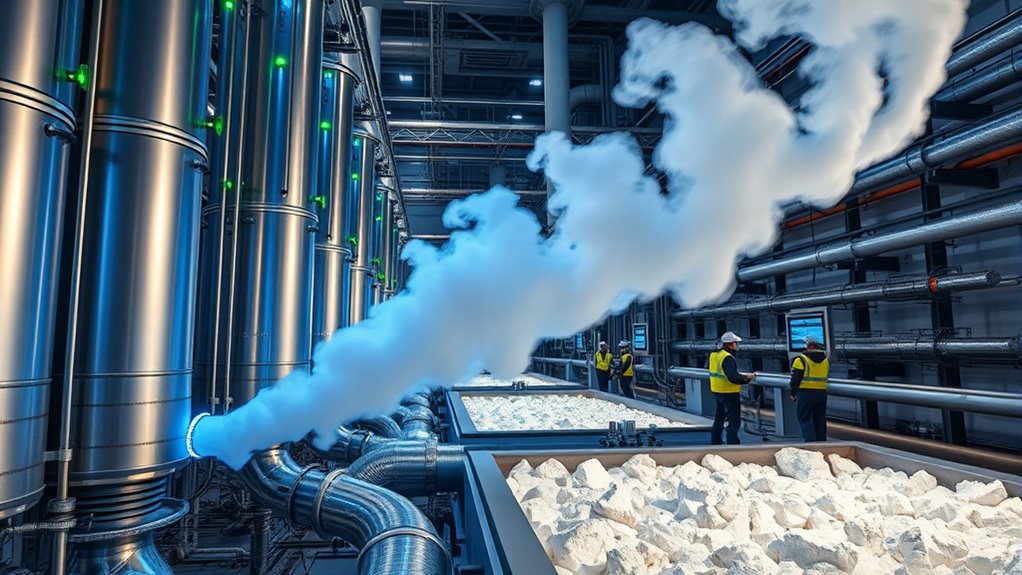
Transforming CO₂ into useful products offers a promising way to turn a greenhouse gas into valuable resources. Electrochemical conversion uses specialized electrodes to produce chemicals like ethylene and formic acid directly from CO₂. Innovations such as tin oxide films and fluorine layers improve selectivity and efficiency, enabling onsite deployment at emission sources like power plants. However, the high energy input required for CO₂-to-CO conversion remains a challenge. These devices convert CO₂ into raw materials for chemicals and fuels, supporting sustainable manufacturing. Companies like Twelve turn captured CO₂ into sustainable aviation fuels, reducing lifecycle emissions by up to 90%. Additionally, molten electrolysis transforms CO₂ into carbon nanotubes and fibers, which are used in lightweight composites for wind turbines and vehicles. These approaches not only recycle CO₂ but also create high-value products, advancing a circular carbon economy.
Comparing the Pros and Cons of Capture Methods
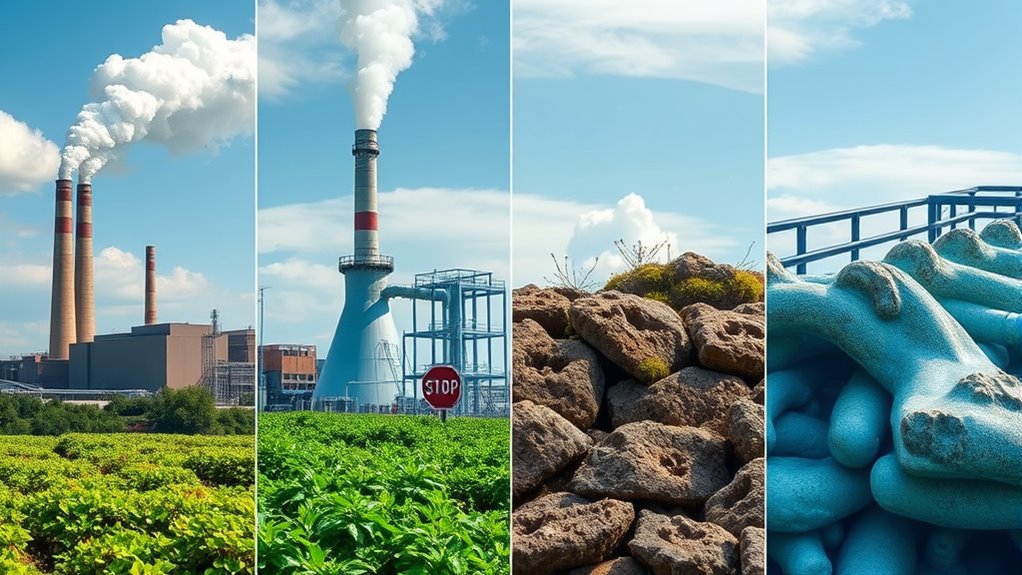
Choosing the right carbon capture method depends on your specific needs and circumstances, as each approach offers distinct advantages and challenges. Post-combustion capture is widely used and compatible with existing infrastructure, but it consumes a lot of energy and incurs high operational costs. Pre-combustion provides nearly complete CO₂ removal and produces hydrogen, yet it requires significant infrastructure changes and is better suited for industrial use. Oxy-fuel combustion simplifies CO₂ separation and can achieve high efficiency, but oxygen production is costly, and safety concerns exist. Direct air capture can remove CO₂ directly from the atmosphere, addressing legacy emissions, but it’s expensive and energy-intensive. Biological methods are low-cost and beneficial for ecosystems but face limitations like land use and slower uptake rates. Each method’s suitability depends on your specific goals and resources. Additionally, incorporating cost-effective solutions such as biological methods can enhance overall sustainability and reduce expenses.
Future Directions in Carbon Capture Technologies
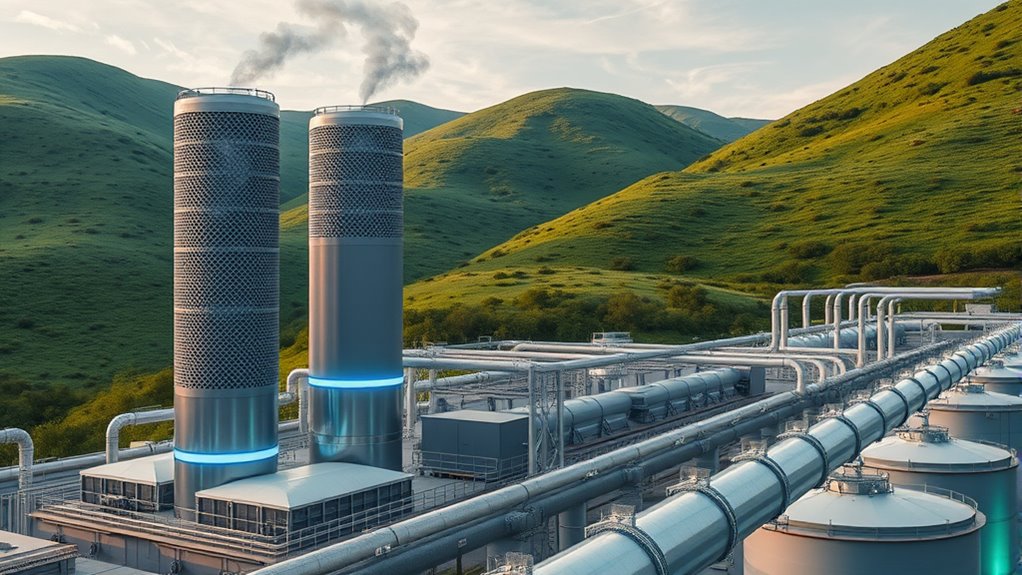
The future of carbon capture technologies is driven by rapid advancements aimed at making processes more efficient, cost-effective, and environmentally sustainable. You’ll see a focus on developing less energy-intensive methods to lower costs and increase adoption.
AI and machine learning will play a key role in optimizing capture and storage processes, boosting overall effectiveness.
Direct Air Capture (DAC) will evolve, but it still needs more renewable energy to be truly sustainable.
Innovation in utilizing captured CO₂—like converting it into plastics or synthetic fuels—will expand, creating new economic opportunities.
Industry players and governments worldwide are investing heavily, especially in Asia Pacific, to build infrastructure and foster international cooperation. These efforts will help meet climate goals while making CCS more accessible and impactful.
Frequently Asked Questions
What Are the Main Costs Associated With Each Carbon Capture Method?
You want to know the main costs for each carbon capture method. For direct air capture, expect high costs, mainly from energy needs and equipment, ranging from $135 to over $1,000 per ton.
Industrial CCS costs vary from $15 to $120 per ton, depending on CO₂ source and technology.
Biological methods are more affordable, often under $20 per ton, mainly involving land management and ongoing maintenance.
How Do Environmental Impacts Vary Among Different Co₂ Capture Technologies?
You might wonder how environmental impacts differ among capture methods. Direct Air Capture reduces atmospheric CO₂ directly with minimal waste but consumes a lot of energy.
Power plant capture effectively cuts emissions but can produce chemical waste.
Post-combustion methods involve solvent use, leading to waste streams.
Oxyfuel combustion emits fewer pollutants and produces pure CO₂ but requires high energy and costs.
Your choice influences environmental benefits and trade-offs.
What Are the Regulatory Challenges Facing Large-Scale Carbon Storage?
You face regulatory challenges like unclear and inconsistent frameworks across countries, which create delays and uncertainties. Existing laws don’t fully address site monitoring, liability, or safety standards, requiring amendments.
Developing suitable infrastructure and transportation networks is costly, adding financial burdens.
Plus, long-term stewardship, risk assessments, and liability concerns demand robust regulation. Political shifts and market uncertainties further complicate project planning, making it essential to establish clear, adaptable regulations for large-scale CO₂ storage success.
How Does Carbon Utilization Contribute to Overall Emission Reductions?
You might wonder how carbon utilization helps cut emissions. It does this by using captured CO₂ in ways that prevent its release, like in enhanced oil recovery or making fuels and chemicals.
This process reduces dependence on fossil fuels, supports a circular economy, and lowers the carbon footprint of industries.
When scaled up, these methods make significant contributions to meeting climate goals and achieving deep decarbonization.
What Innovations Are Expected to Lower Costs of Future Carbon Capture Solutions?
You see innovation shaping the future of carbon capture, reducing costs through advancements like improved membranes, modular systems, and scalable designs. These innovations streamline deployment, cut land use, and enhance efficiency.
Breakthrough materials, such as MOFs and advanced solvents, boost capture capacity. As these technologies mature, costs drop, capacities rise, and applications expand, making carbon capture more affordable and widespread—helping you envision a cleaner, more sustainable world with each breakthrough.
Conclusion
Now that you’ve seen how each method traps CO₂ like a net catching escaping fish, you’re better equipped to understand the future of clean air. From direct air capture to turning CO₂ into useful products, these technologies are shaping a greener world. While no single solution is perfect, working together, they form a tapestry of hope. Keep pushing for innovation—because every effort is a step toward clearing the air we all breathe.