When you dissolve salt in water, it dissociates into charged particles called ions, mainly positive cations and negative anions. These ions move freely and carry electrical charge, allowing electricity to flow through the solution. The more ions present, the better the solution conducts electricity. Understanding how salts ionize and their concentration influence conductivity helps explain why salt solutions are good conductors. Explore further to see how different factors affect this process and its real-world applications.
Key Takeaways
- Salt solutions conduct electricity because they dissociate into ions that carry charge.
- Ions move in response to electric fields, creating an electrical current in the solution.
- The more ions present, the higher the solution’s electrical conductivity.
- Complete dissociation of salts like NaCl results in strong electrolytes with high conductivity.
- The movement of positive and negative ions enables salt solutions to effectively transmit electrical energy.
What Are Electrolytes and How Do They Work?
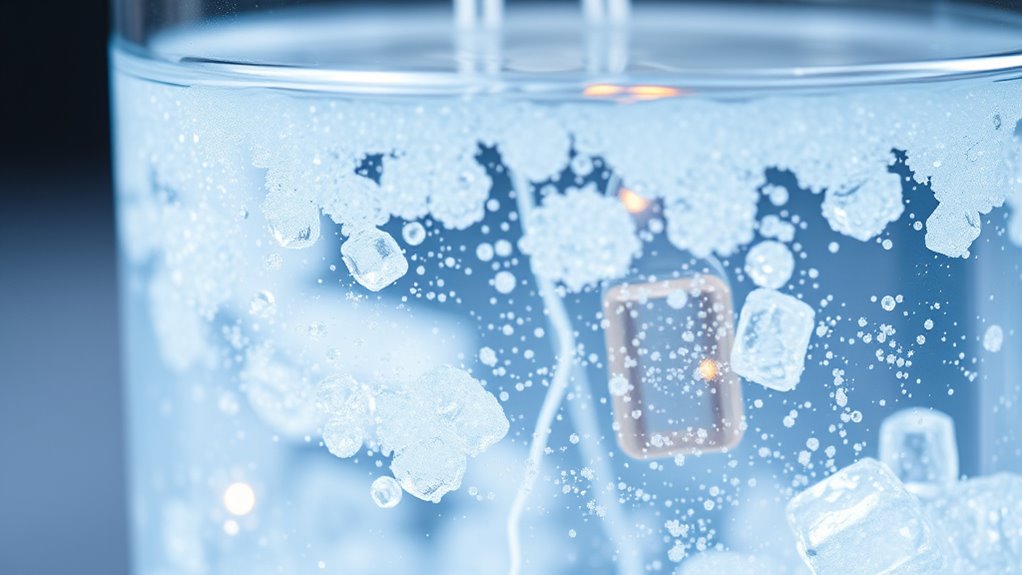
Electrolytes are substances that carry an electric charge and conduct electricity when dissolved in water, making them vital for many bodily functions.
Electrolytes carry electric charges and are essential for powering your body’s vital functions.
When you dissolve electrolytes like sodium or potassium in water, they dissociate into charged particles called ions—positively or negatively charged. These ions move freely in the solution, allowing it to conduct electricity.
This movement enables electrical signals in your body to travel efficiently, supporting nerve impulses and muscle contractions. The ions also help balance the electrical charge within your cells and fluids.
Maintaining proper electrolyte levels is essential for overall health and ensures your body’s physiological functions stay in balance. Additionally, the process of dissociation in electrolytes is crucial for their ability to conduct electricity effectively.
Types of Electrolytes Found in Salt Solutions
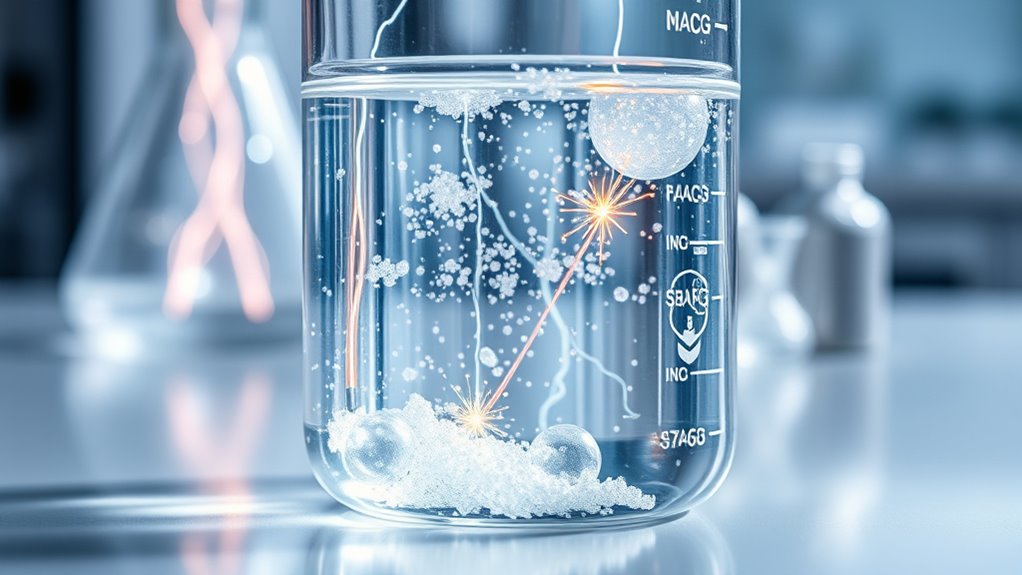
In salt solutions, ions are classified based on their electrical charges, with cations carrying positive charges and anions carrying negative ones. Common cations include sodium (Na⁺), potassium (K⁺), calcium (Ca²⁺), and magnesium (Mg²⁺).
Typical anions are chloride (Cl⁻), phosphate (PO₄³⁻), bicarbonate (HCO₃⁻), and sulfate (SO₄²⁻).
Electrolytes can be strong or weak, depending on how completely they dissociate into ions. Strong electrolytes like NaCl and KCl fully dissociate, leading to high conductivity.
Weak electrolytes, such as acetic acid, dissociate partially, resulting in fewer free ions and lower conductivity.
The ionic composition influences both the solution’s ability to conduct electricity and its biological and chemical functions. Understanding ion dissociation is essential for comprehending how different electrolytes behave in various environments.
How Ionization Enables Electricity Conduction
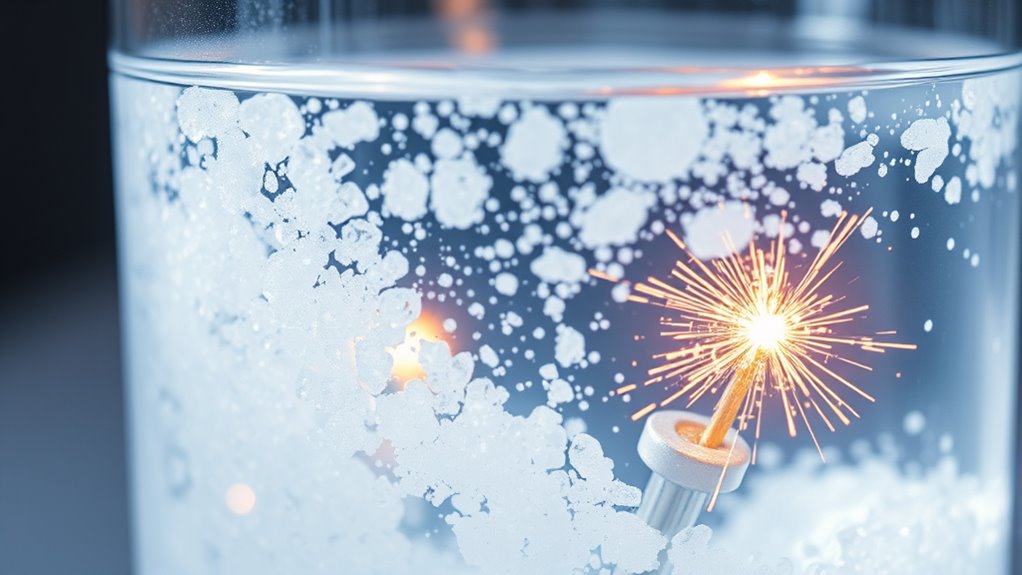
How does electricity travel through a solution? It happens when ions move in response to an electric field, carrying electrical charges. In electrolytes, full atomic species—ions—are what move, not molecules, allowing current to flow. The concentration of these ions impacts conductivity: more ions mean better conduction. When an electric field is applied, ions drift toward oppositely charged electrodes, creating a current. Their drift velocity depends on the electric field strength and ion mass. Higher ion concentrations lead to lower resistance, enabling electricity to pass more easily. Fundamentally, ionization transforms dissolved substances into charged particles that respond to electric forces, making solutions like saltwater good conductors. Understanding credit card data protection is crucial for maintaining data security in payment processing. This ion movement is the core reason why electrolytes can carry electrical current efficiently.
Measuring Conductivity in Saltwater
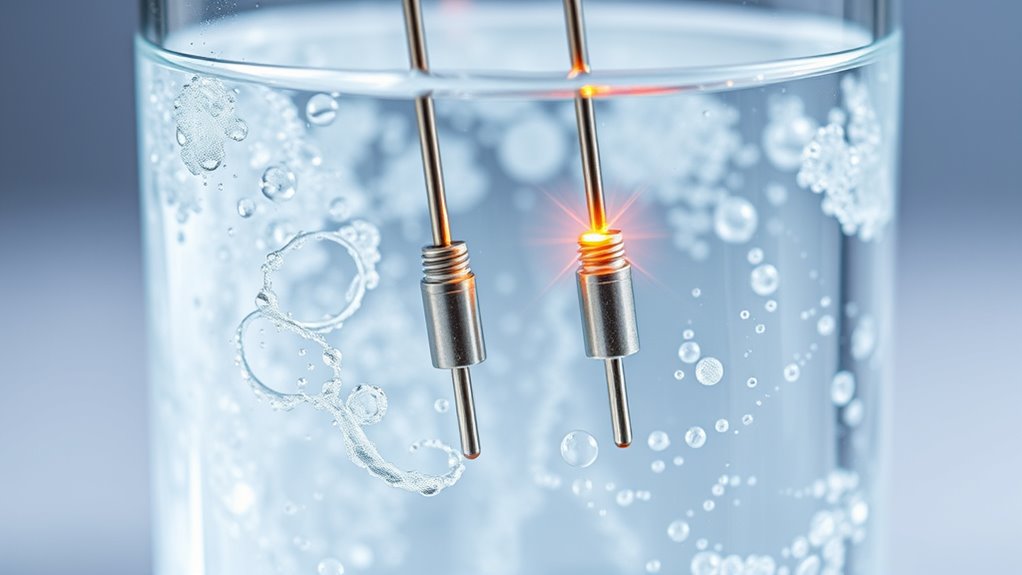
Measuring conductivity in saltwater involves passing a small electric current between electrodes submerged in the sample and observing how easily the current flows. You’ll use a conductivity meter, which applies current through corrosion-resistant electrodes, often titanium coated with platinum black, to guarantee durability and accuracy. Many pocket meters, like the LAQUAtwin Salt 11, come pre-calibrated with standard seawater and NaCl solutions, simplifying your process. Digital meters, such as the ATAGO ES-421, measure salinity directly using conductivity, requiring only about 1 mL of sample. Regular calibration with known NaCl standards at different concentrations assures dependable results. Conductivity is expressed in microsiemens per centimeter (µS/cm) or millisiemens per centimeter (mS/cm), reflecting ionic concentration. Temperature compensation is essential, as conductivity varies with temperature changes. Understanding the influence of temperature on conductivity is crucial for accurate measurements, especially in varying environmental conditions.
The Role of Concentration in Conductivity
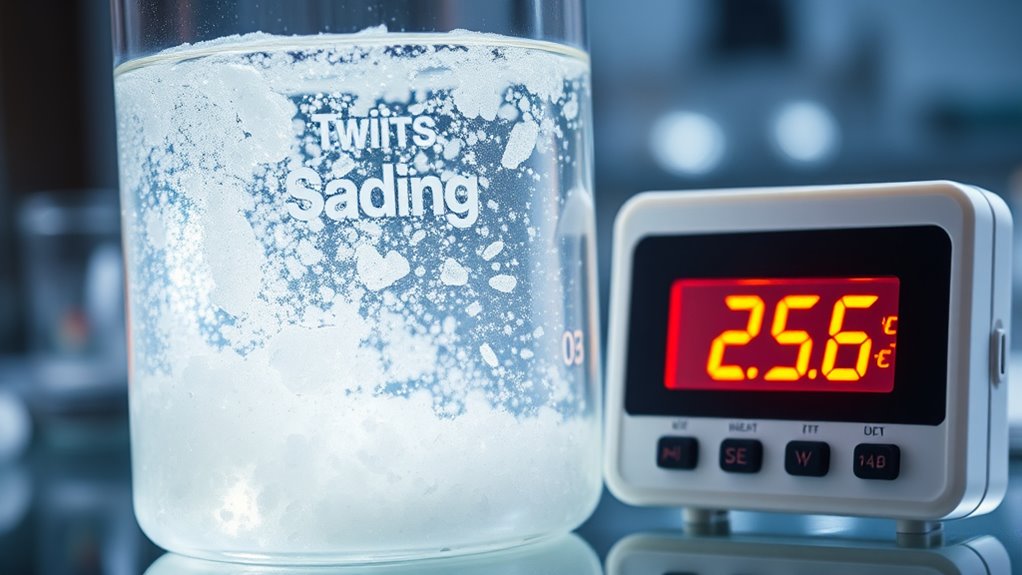
Concentration plays a key role in determining a solution’s conductivity because it directly influences the number of charge carriers present. As you increase ion concentration, conductivity generally rises since more ions are available to carry charge. Additionally, recent advances in machine learning algorithms are being utilized to model and predict complex relationships between ion concentration and conductivity more accurately. However, this relationship isn’t always linear; at high concentrations, ions can interact and form associations, reducing the number of free charge carriers. Ion mobility may decrease with concentration, but the overall conductivity often still increases because of the higher ion count. Additionally, different solvents and electrolyte types can alter how concentration affects conductivity. Beyond certain limits, adding more ions doesn’t considerably boost conductivity due to crowding effects.
Understanding this balance helps optimize solutions for electrochemical applications where precise conductivity control is essential.
Differences Between Strong and Weak Electrolytes
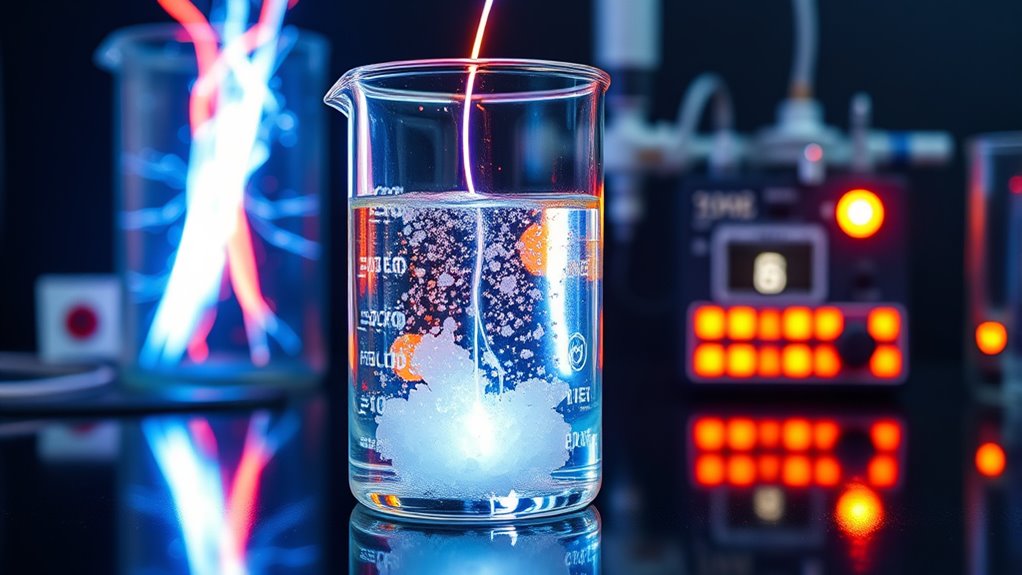
The key difference between strong and weak electrolytes lies in their extent of ionization when dissolved in water. Strong electrolytes ionize completely, nearly 100%, producing plenty of free ions that conduct electricity efficiently. Common examples include strong acids like HCl, strong bases like NaOH, and soluble salts such as NaCl. This complete ionization allows for maximum electrical conductivity. In contrast, weak electrolytes only partially ionize, usually about 1–10%, so fewer ions are available. Weak acids like acetic acid and weak bases like ammonia fall into this category. The degree of ionization directly influences conductivity: strong electrolytes conduct electricity well, producing bright light in a conductivity test, while weak electrolytes conduct poorly, resulting in dim light. Recognizing these differences helps predict how solutions will behave in various chemical contexts.
Biological and Environmental Significance of Electrolytes
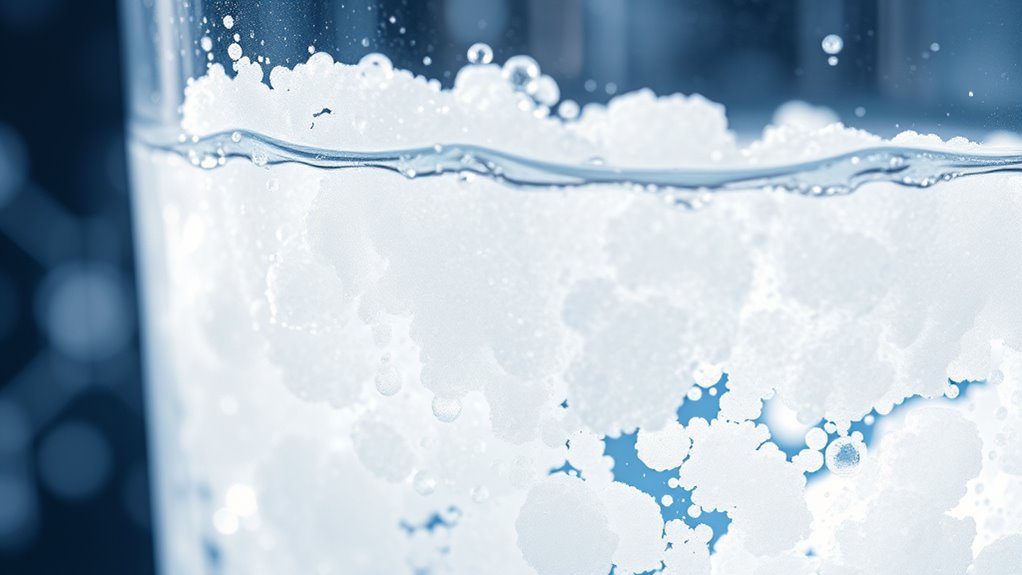
Have you ever wondered how electrolytes play a crucial role in both living organisms and the environment? In your body, electrolytes regulate fluid balance, ensuring water is properly distributed across cells and tissues. They enable nerve impulses, with sodium and potassium managing electrical signals essential for communication.
Electrolytes like calcium and magnesium assist muscles in contracting and relaxing. They also help maintain pH balance through bicarbonate, supporting overall health.
Environmentally, electrolytes make solutions conductive, aiding in industrial processes, water treatment, and energy storage in batteries. They influence soil fertility by providing essential nutrients like potassium and magnesium, which support plant growth.
Understanding the cost of building a tiny house community and its infrastructure needs can help in planning sustainable environments for multiple residents. However, excess electrolytes can pollute water, harm ecosystems, and impact human health, highlighting their vital yet sensitive role in our world.
Techniques for Analyzing Electrolyte Solutions
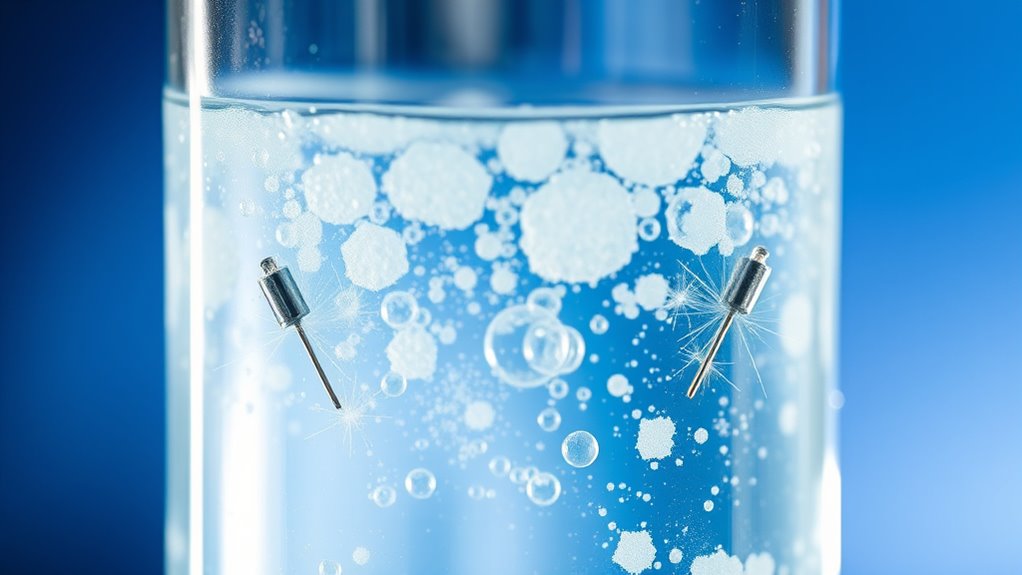
Analyzing electrolyte solutions requires a combination of techniques that reveal their thermal, rheological, and compositional properties. Differential Scanning Calorimetry (DSC) helps you measure melting points, crystallization, and thermal stability, which are essential for safety assessments. Remote work has shown that flexible and efficient analysis methods can improve productivity in laboratory settings, especially when resources are limited.
Electrolyte analysis combines thermal, rheological, and compositional techniques for comprehensive insight.
Thermogravimetric Analysis (TGA) tracks weight changes during heating to identify decomposition temperatures.
Rheology measures viscosity and flow behavior, informing you about pumpability and stability under operational conditions.
Conductivity tests reveal how efficiently ions move within the solution, crucial for battery design.
Ion chromatography (IC) provides precise ion concentrations, detecting impurities or degradation products.
When combined, these methods give a thorough profile of electrolyte behavior, ensuring peak performance, safety, and longevity in various applications.
Controlled environments like argon or nitrogen are often necessary to prevent contamination during testing.
Practical Uses of Electrolyte Conductivity Data
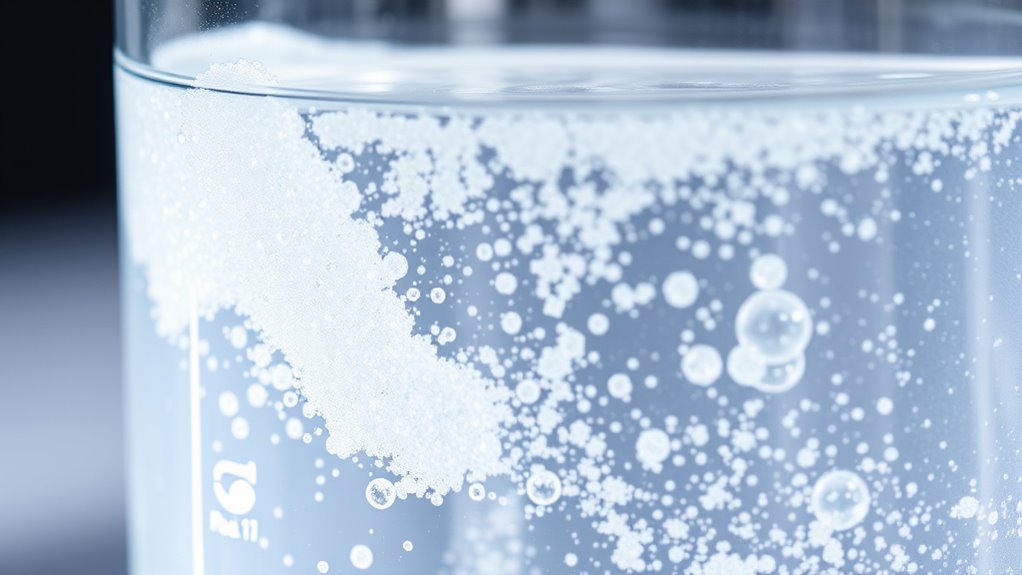
Electrolyte conductivity data play a crucial role in various industrial and scientific applications by providing real-time insights into solution quality and system performance. In industries like water treatment and chemical processing, conductivity helps detect dissolved solids buildup, preventing scaling and maintaining efficiency.
It’s essential for monitoring salt brine strength in food preservation and ensuring consistent product quality. Conductivity also influences battery performance, affecting charging rates and overall efficiency, especially in lithium-ion systems.
In fuel cells and electroplating, conductivity data optimize power output and metal deposit quality. Regular checks prevent equipment scaling, support predictive maintenance, and improve reaction yields in chemical reactors.
Additionally, conductivity aids scientific research—studying ocean currents, soil salinity, and groundwater—driving innovations in new battery, desalination, and bioelectrochemical technologies.
Frequently Asked Questions
How Do Temperature Changes Affect Electrolyte Conductivity?
When temperature changes, you’ll notice electrolyte conductivity adjusts accordingly.
As temperature rises, ions gain more kinetic energy, move faster, and dissociate more, increasing conductivity.
Conversely, lower temperatures cause ions to slow down, reducing ionization and making conductivity drop.
You should also consider the electrolyte’s temperature coefficient, which indicates how sensitive it’s to temperature shifts.
Can Non-Electrolyte Solutions Ever Conduct Electricity?
You might wonder if non-electrolyte solutions can ever conduct electricity. Generally, they don’t because their molecules stay intact and don’t produce free ions needed for conduction.
However, impurities or the solvent’s autoionization, like water producing H+ and OH- ions, can cause very slight conductivity.
Still, in pure form, non-electrolyte solutions act as insulators, with minimal or no electrical current passing through.
What Determines Whether an Electrolyte Is Classified as Strong or Weak?
You determine whether an electrolyte is classified as strong or weak based on how completely it ionizes in water. Strong electrolytes fully dissociate into ions, making them excellent conductors of electricity.
Weak electrolytes only partially dissociate, resulting in limited ion presence and poorer conductivity. Factors like solubility, chemical nature, and ionization degree influence this classification.
This classification directly affects their ability to conduct electricity in solution.
How Do Electrolytes Influence the Ph of a Solution?
You might wonder how electrolytes influence the pH of a solution. When electrolytes dissolve, they release ions that can either donate or accept hydrogen ions, directly affecting pH levels.
For example, acids increase hydrogen ions, lowering pH, while bases release hydroxide ions, raising pH. Your body’s balance depends on these interactions, which regulate acidity and alkalinity, ensuring proper physiological functions.
Are There Environmental Risks Associated With Electrolyte Runoff?
You might overlook electrolyte runoff as harmless, but it’s a potential environmental disaster waiting to happen. It can flood water bodies with excess salt, killing aquatic life and disrupting ecosystems faster than you’d think.
Toxic chemicals like PFAS and heavy metals can leach into soil and water, causing long-term damage. Proper management and disposal are vital, or you risk releasing chaos on your local environment that’s hard to undo.
Conclusion
Understanding electrolytes helps you appreciate how salt solutions conduct electricity. By grasping ionization and concentration effects, you see their importance in daily life and nature. Remember, “the more you know, the better you grow,” so keep exploring how electrolytes impact health, environment, and technology. Staying curious about these tiny particles reveals big secrets—sometimes, the simplest solutions hold the greatest power.