Intermolecular forces include dispersion, dipole-dipole, and hydrogen bonds. Dispersion forces are weak and occur in all molecules, especially larger ones, due to temporary electron movements. Dipole-dipole interactions happen between molecules with permanent poles, affecting properties like boiling points. Hydrogen bonds are strong dipole attractions involving N, O, or F and hydrogen, vital in biological structures. Understanding these forces helps explain material behaviors and biological functions, and you’ll discover more about their effects as you explore further.
Key Takeaways
- London dispersion forces are the weakest, present in all molecules, caused by temporary electron fluctuations creating brief dipoles.
- Dipole-dipole interactions occur between molecules with permanent positive and negative regions, influencing their physical properties.
- Hydrogen bonding requires hydrogen attached to N, O, or F, resulting in strong, directional intermolecular attractions.
- The strength of these forces affects boiling points, solubility, and molecular stability across different substances.
- Larger molecules and increased polarity generally lead to stronger dispersion and dipole interactions, impacting material and biological behaviors.
Understanding London Dispersion Forces
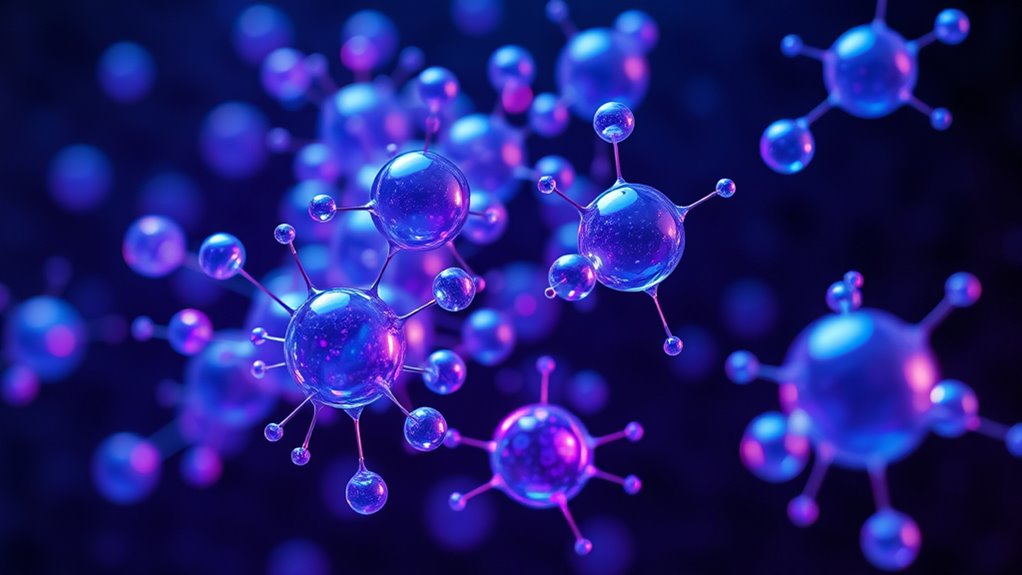
London dispersion forces are the weakest type of intermolecular forces, but they’re present in all molecules, whether polar or nonpolar. You mightn’t notice them directly, but they’re caused by temporary dipoles that form as electrons move around atoms or molecules. These fluctuations create uneven electron distributions, leading to short-lived dipoles that induce similar dipoles in nearby molecules. The result is a weak electrostatic attraction between these temporary dipoles. Although individually small, these forces become significant in bulk materials because their cumulative effect can influence physical properties like boiling and melting points. They are especially important for nonpolar substances, such as hydrocarbons, where they help explain phenomena like condensation and solidification at low temperatures. London dispersion forces are fundamental to understanding the behavior of nonpolar molecules in various states of matter. Additionally, they play a crucial role in molecular interactions, which are essential for the physical characteristics of many substances.
The Nature of Dipole-Dipole Interactions
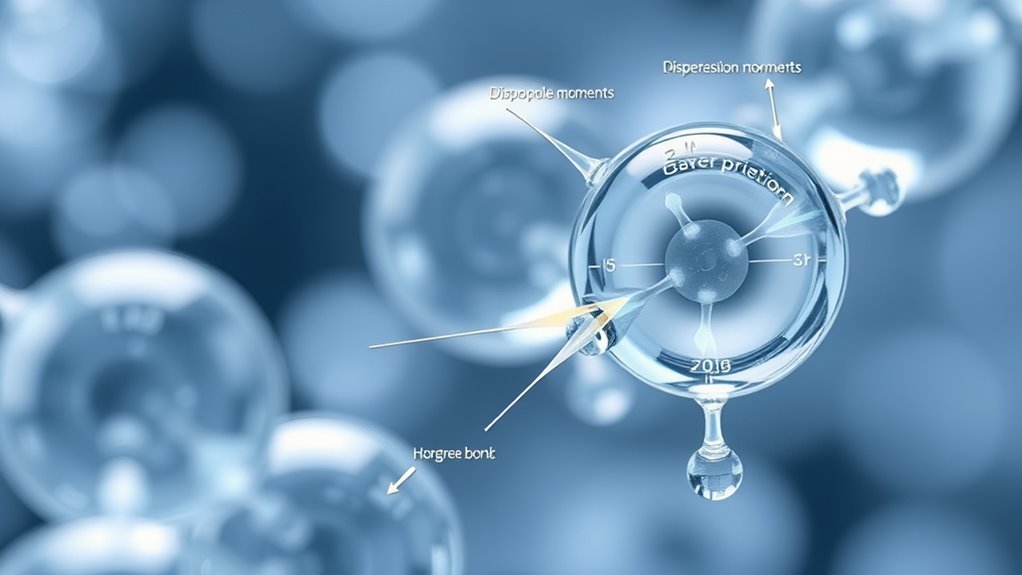
Dipole-dipole interactions occur between molecules that have permanent electric dipoles, meaning they possess regions with partial positive and negative charges. These forces arise from the attraction between the positive end of one molecule and the negative end of another. The strength of these interactions depends on molecular structure and how polar the molecules are. Molecules like water and sulfur dioxide exhibit dipole-dipole forces, which influence their physical properties, such as boiling points and viscosity. These interactions are directional, so the molecules’ orientation affects their strength. Generally, dipole-dipole interactions are stronger than London dispersion forces but weaker than hydrogen bonds. They play a key role in biological systems, affecting how molecules recognize each other and how substances behave in different environments. Understanding the molecular polarity is essential to grasp how these forces operate effectively in various contexts. Additionally, the polar nature of molecules influences their miscibility and solubility in different solvents, further impacting their behavior.
What Makes Hydrogen Bonding Unique
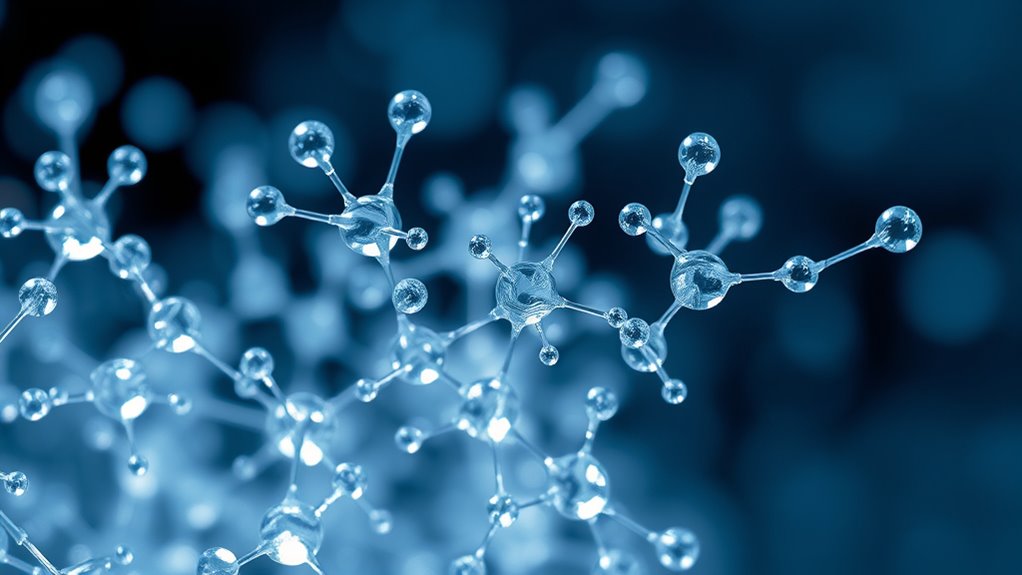
What makes hydrogen bonding truly unique is its strict electronic and structural requirements. You need hydrogen covalently bonded to highly electronegative atoms like nitrogen, oxygen, or fluorine. These atoms pull electron density away from hydrogen, creating a significant partial positive charge. They also have lone pairs of electrons in low-energy orbitals, which are highly effective at attracting hydrogen’s positive charge. This setup makes hydrogen almost like a bare proton, enabling strong dipole attractions. Lone pairs of electrons are crucial for the formation of hydrogen bonds because they act as electron donors in the interaction. Unlike other dipole-dipole interactions, hydrogen bonds are especially stronger and highly directional. They influence molecular geometry, promote network formation, and impact physical properties like melting points, boiling points, and water’s unique characteristics. The directionality of hydrogen bonds is vital for their biological significance, such as in DNA structure. Additionally, the unique electronic configuration of the involved atoms enhances the strength and specificity of these bonds. These specific conditions explain why hydrogen bonding plays such an essential role in chemistry and biology.
Comparing the Strength of Intermolecular Forces
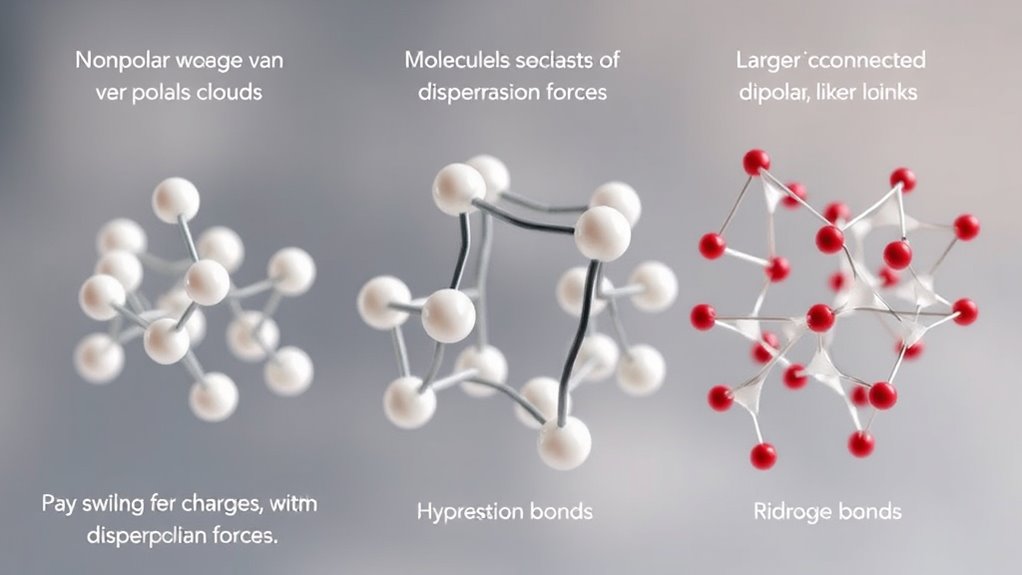
Intermolecular forces vary considerably in strength, directly affecting a substance’s physical properties such as melting and boiling points. You’ll find that London dispersion forces are the weakest, present in all molecules, especially nonpolar ones like methane. Dipole-dipole forces are stronger, occurring in polar molecules where partial charges attract. Hydrogen bonds are the strongest intermolecular forces after ionic bonds, involving hydrogen bonded to electronegative atoms like oxygen or nitrogen, as seen in water and ammonia. The Crochet Styles for Locs offer a creative way to explore styling techniques, just as understanding different intermolecular forces can help explain the diverse physical properties of substances. When comparing compounds, ionic substances have the strongest forces, resulting in high melting points. Polar molecules with hydrogen bonding tend to have higher boiling points than those with only dipole-dipole or dispersion forces. The strength of these forces directly correlates with the energy needed to break them.
How Forces Influence Physical States and Boiling Points
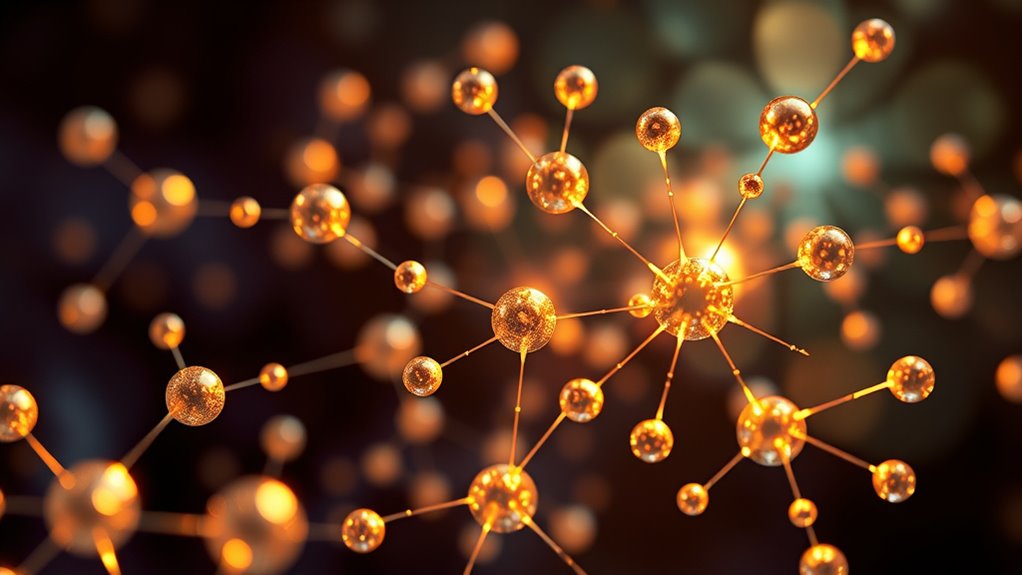
Have you ever wondered why some substances boil at much higher temperatures than others? The answer lies in the strength of their intermolecular forces. Dispersion forces grow stronger with larger molecules and greater surface area, raising boiling points.
Intermolecular forces determine boiling points; larger molecules and greater surface area lead to higher temperatures.
Dipole-dipole interactions depend on molecular polarity and shape; more polar molecules need more energy to overcome these forces. Hydrogen bonding, being particularly strong, considerably elevates boiling points compared to other forces.
Stronger intermolecular forces reduce vapor pressure, meaning higher temperatures are necessary for boiling. Molecules with high polarity, larger surface areas, or the ability to hydrogen bond tend to have higher boiling points.
These forces keep molecules together in the liquid state and influence how much energy is required for phase changes.
The Role of Molecular Size and Polarity
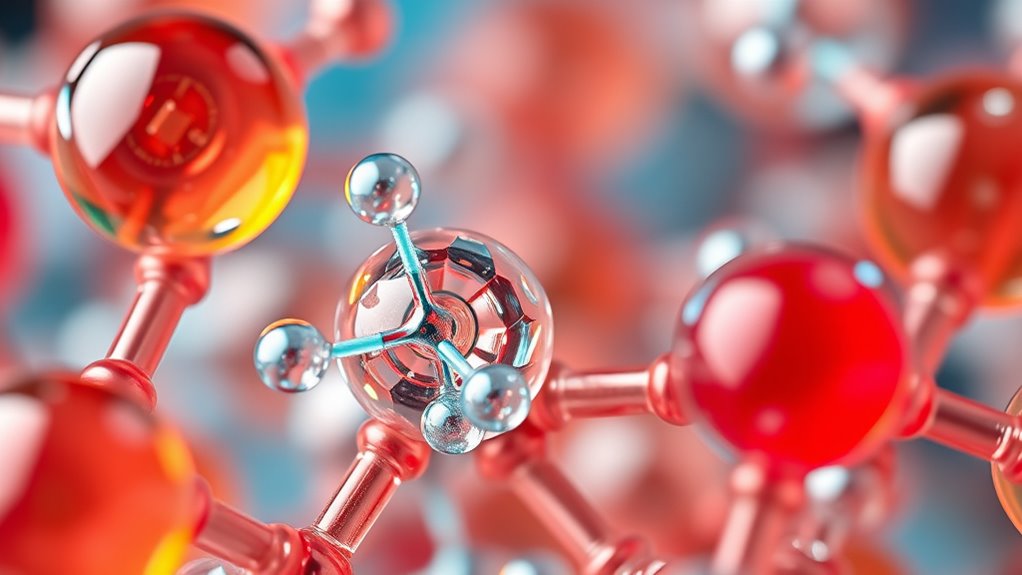
Molecular size and polarity play crucial roles in determining the strength of intermolecular forces. Larger molecules have more electrons and surface area, boosting London dispersion forces, even in nonpolar substances. For example, octane’s higher boiling point compared to smaller alkanes results from stronger dispersion forces.
Conversely, polarity introduces dipole-dipole interactions, where partial charges attract, increasing overall intermolecular strength. Polar molecules with substantial dipoles exhibit stronger attractions than similar-sized nonpolar molecules.
When size and polarity combine, as in larger polar molecules, the effects amplify, leading to higher boiling points and better solubility.
Shape also influences these forces; asymmetrical molecules create net dipoles, enhancing interactions. Overall, both size and polarity shape the strength and type of intermolecular forces, impacting physical properties profoundly.
Significance in Biological Systems and Materials
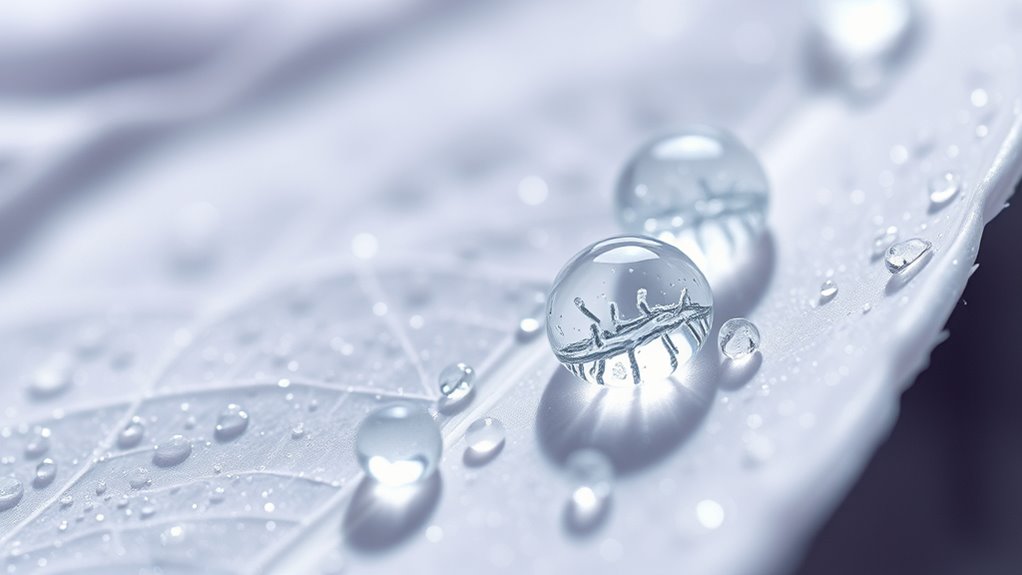
Why do biological systems rely so heavily on subtle forces like dispersion interactions? These forces occur between all molecules, influencing how molecules pack and behave in cells and tissues. They help stabilize hydrophobic interactions crucial for protein folding and membrane formation.
As the size of molecules’ electron clouds increases, dispersion forces grow stronger, affecting how large biomolecules interact and assemble. These weak forces also support the stability of protein structures by providing subtle attractions within hydrophobic cores.
Additionally, dispersion interactions enable nonpolar molecules like lipids to aggregate, forming cell membranes and organelles. Understanding these forces reveals how biological systems maintain structure and function at a molecular level, highlighting the importance of even the faintest attractions in complex life processes and material properties.
Practical Implications in Industry and Chemistry
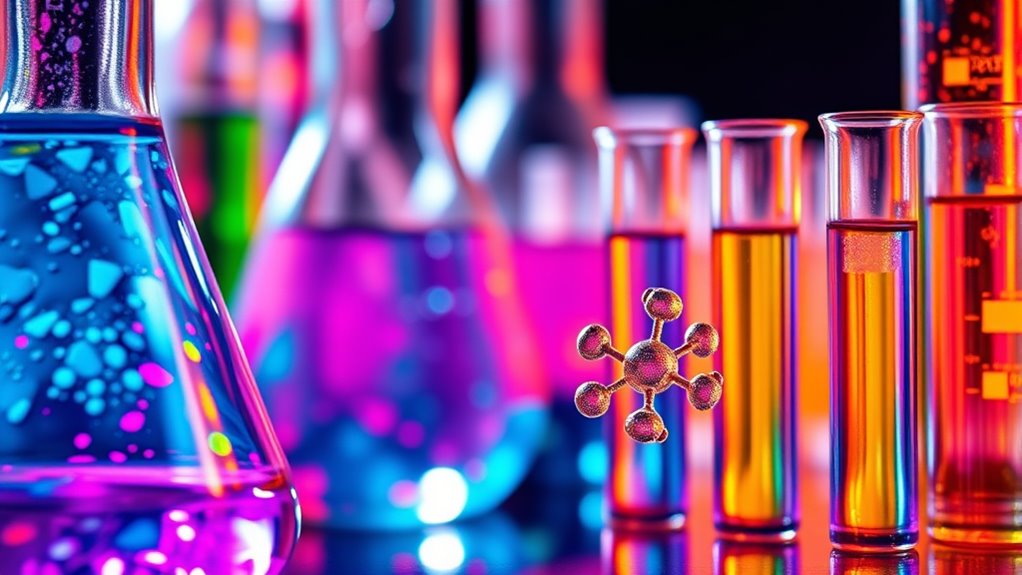
Intermolecular forces play a crucial role in shaping the properties and performance of materials across various industries. In material engineering, these forces determine polymer flexibility, durability, and rigidity.
For example, polyethylene’s flexibility comes from London dispersion forces, making it suitable for packaging, while PVC’s stronger dipole-dipole interactions provide rigidity for pipes and cables. Construction materials rely on ion-ion, hydrogen bonding, and dipole forces to achieve strength and durability, especially in composites.
In aerospace, bonding techniques that optimize intermolecular forces reduce weight and enhance strength—about 30% of Airbus components are bonded instead of fastened.
In pharmaceuticals, intermolecular interactions influence drug solubility and bioavailability, guiding formulation and delivery strategies. Understanding and manipulating these forces enable industry innovations and improve material performance.
Visualizing Intermolecular Attractions and Effects
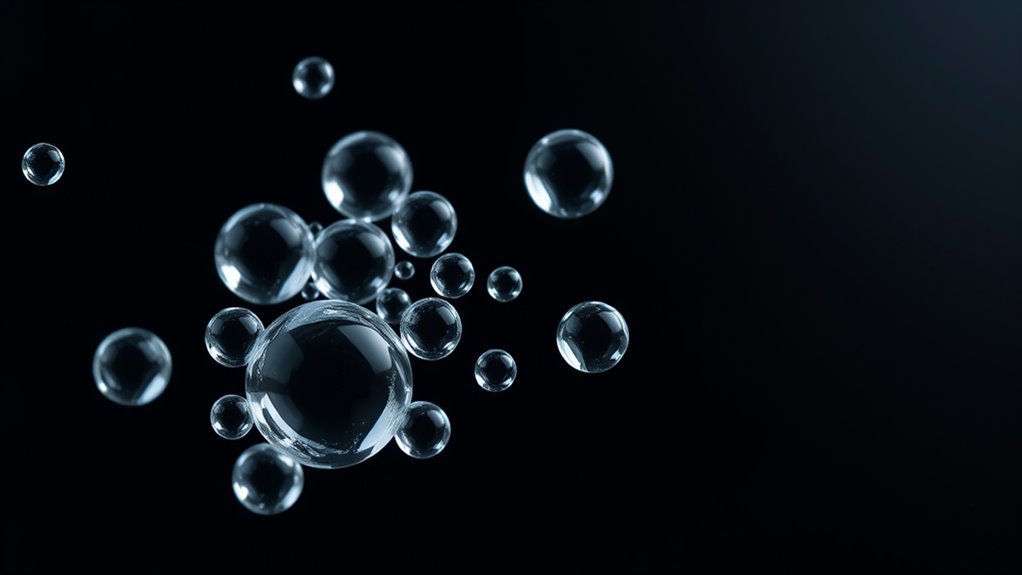
Visualizing intermolecular attractions helps you grasp how molecules interact and influence material properties. Tactile models made from inexpensive materials let you physically explore hydrogen bonding, dipole–dipole forces, and London dispersion forces, enhancing multisensory learning. These models make complex concepts clearer by allowing hands-on comparison and active review.
Computer simulations take visualization further by showing molecular behavior, motion, and alignment dynamically, helping you see how forces affect states and phase changes. 3D models and simulations of molecular geometry reveal how polarity and shape influence interactions and bonding possibilities.
Macroscopic properties like boiling points, viscosity, and vapor pressure serve as visual indicators of force strength, while quantum and computational tools illustrate forces at the electronic level. All these methods deepen your understanding of intermolecular attractions.
Frequently Asked Questions
How Does Temperature Affect the Strength of Intermolecular Forces?
When you increase temperature, you weaken the strength of intermolecular forces. This happens because higher temperatures boost the particles’ kinetic energy, making it harder for them to stay close together.
As particles move faster, they break free from these attractions more easily, which leads to phase changes like melting or boiling.
Can Intermolecular Forces Influence Solubility in Different Solvents?
Think of intermolecular forces as bridges connecting molecules, shaping whether they can mix in different solvents. When these forces are strong, molecules tend to stay together, making solubility in certain solvents easier if the forces match.
If they’re weak or mismatched, molecules may refuse to mingle. So, yes, intermolecular forces influence how well substances dissolve, guiding them to find compatible partners in various solvents for a perfect blend.
Why Do Substances With Similar Molecular Weights Have Different Boiling Points?
You wonder why substances with similar molecular weights have different boiling points. It’s because factors like polarity, hydrogen bonding, molecular shape, and functional groups play a vital role.
If a molecule can form hydrogen bonds or has a polar structure, it will have a higher boiling point than a nonpolar or less interactive molecule.
Shape and surface area also affect dispersion forces, influencing boiling points beyond just molecular weight.
How Do Hydrogen Bonds Impact the 3D Structure of Proteins?
Hydrogen bonds critically influence the 3D structure of proteins by stabilizing their secondary and tertiary formations. They form between backbone groups, creating alpha helices and beta sheets.
They also occur between side chains, shaping the overall fold. These bonds orient and lock the protein into its functional shape, especially when buried inside the molecule.
Disrupting hydrogen bonds can lead to unfolding or misfolding, highlighting their essential role in maintaining structural integrity.
Are Intermolecular Forces Responsible for the Condensation of Gases Into Liquids?
Think of gas molecules as dancers on a crowded floor, moving freely until they start to feel the pull of each other’s attraction. Yes, intermolecular forces are responsible for gas condensation into liquids.
When these attractive forces outweigh the molecules’ kinetic energy, they come together, forming a liquid. This process releases energy and depends on the strength of these forces, which control the ease of condensation and the properties of the resulting liquid.
Conclusion
Understanding intermolecular forces is like revealing nature’s invisible glue that holds molecules together. By grasping how dispersion, dipole, and hydrogen bonds work, you see how they shape everything from boiling points to biological functions. These forces are the unseen puppeteers behind physical states and material properties. Once you see their influence, you’ll realize they’re the silent architects of the world around you, quietly shaping life and matter in a dance as intricate as a spider’s web.