Allotropes are different forms of the same element with unique structures, like carbon’s diamond and graphite. You’ll find that their arrangement of atoms causes significant differences in hardness, electrical conductivity, and chemical reactivity. Diamond has a 3D network of strong covalent bonds, making it very hard and insulating, while graphite has layers that slide easily, making it soft and conductive. To understand why these forms differ so much, keep exploring their structures and properties.
Key Takeaways
- Allotropes are different structural forms of the same element, caused by variations in atomic arrangements.
- Diamond’s atoms form a 3D tetrahedral network, making it extremely hard and insulating.
- Graphite consists of layered 2D hexagonal sheets with weak van der Waals forces, making it soft and conductive.
- The different bonding types (sp³ in diamond, sp² in graphite) lead to distinct physical and chemical properties.
- Variations in structure and bonding explain why diamond and graphite have contrasting hardness, conductivity, and reactivity.
Understanding Allotropes and Their Significance
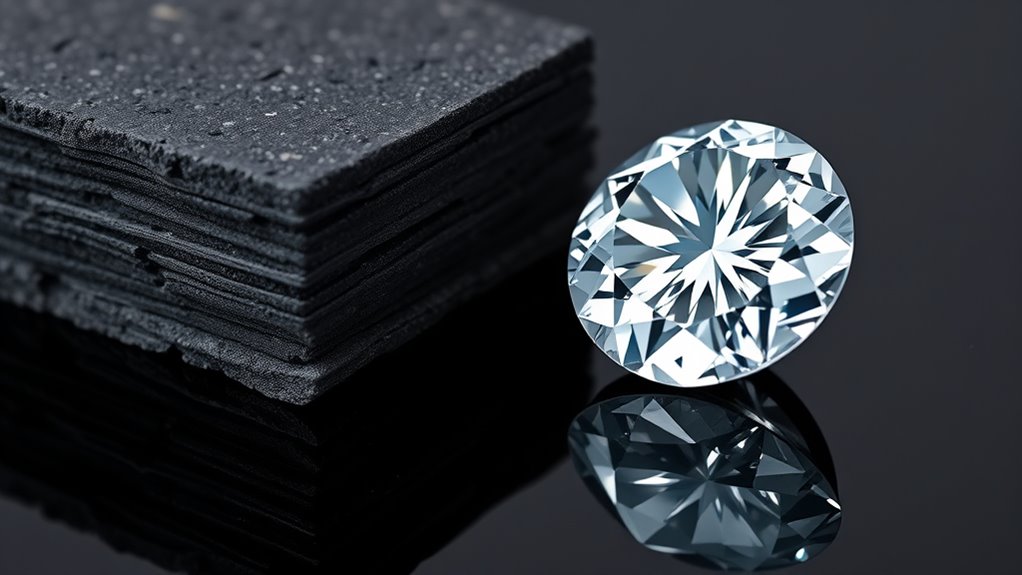
Allotropes are different forms of the same element that arise from variations in atomic arrangements. As you explore these forms, you’ll notice they only occur in specific elements like carbon, oxygen, phosphorus, sulfur, and tin. These variations influence their physical and chemical properties, making each allotrope uniquely suited for certain applications. For example, diamond and graphite are both carbon allotropes, but their structures give them vastly different hardness, conductivity, and uses. Understanding how atoms connect and the resulting molecular structure is crucial to comprehending why these allotropes behave so differently. Understanding allotropy helps you grasp how the same element can behave so differently under various conditions. Recognizing these differences also highlights their significance in technology, research, and environmental processes.
The Distinct Structures of Carbon Allotropes
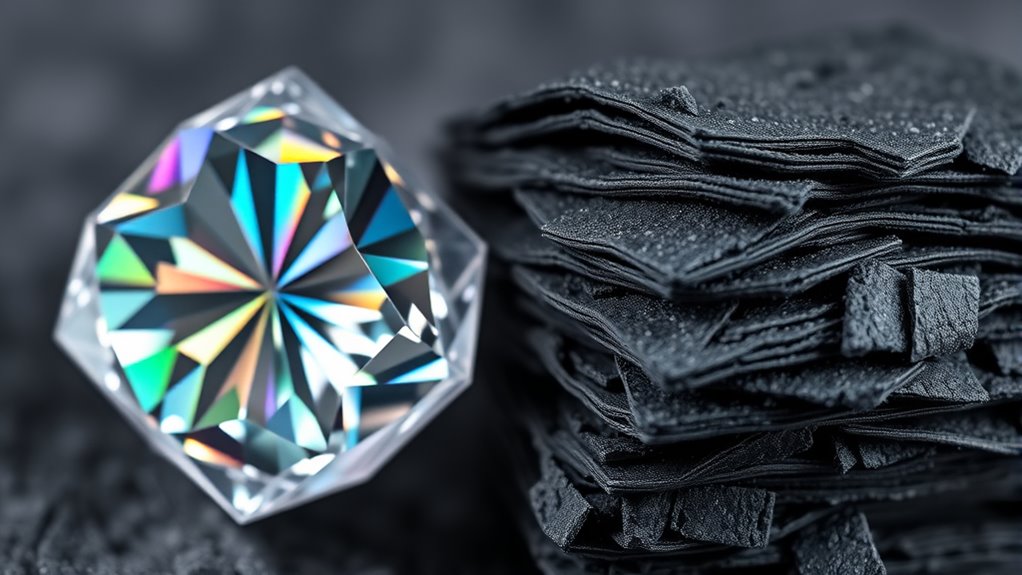
The unique structures of carbon allotropes arise from how their atoms are arranged at the microscopic level. In diamond, atoms form a three-dimensional tetrahedral lattice, with each carbon bonded to four neighbors at equal angles. This creates a rigid, strong network extending in all directions. This tetrahedral bonding results in diamond’s exceptional hardness. In contrast, graphite consists of flat, two-dimensional sheets of hexagonal rings bonded by three covalent bonds. These layers are stacked in an ABAB sequence and held together by weak van der Waals forces, allowing them to slide easily. The carbon atoms in graphite are sp² hybridized, forming delocalized π bonds above and below the layers, which enable electrical conductivity. These differences in atomic arrangement and bonding define the distinct physical and chemical properties of diamond and graphite. Additionally, the bonding types present in each allotrope influence their thermal and electrical behaviors.
Comparing the Physical Properties of Diamond and Graphite
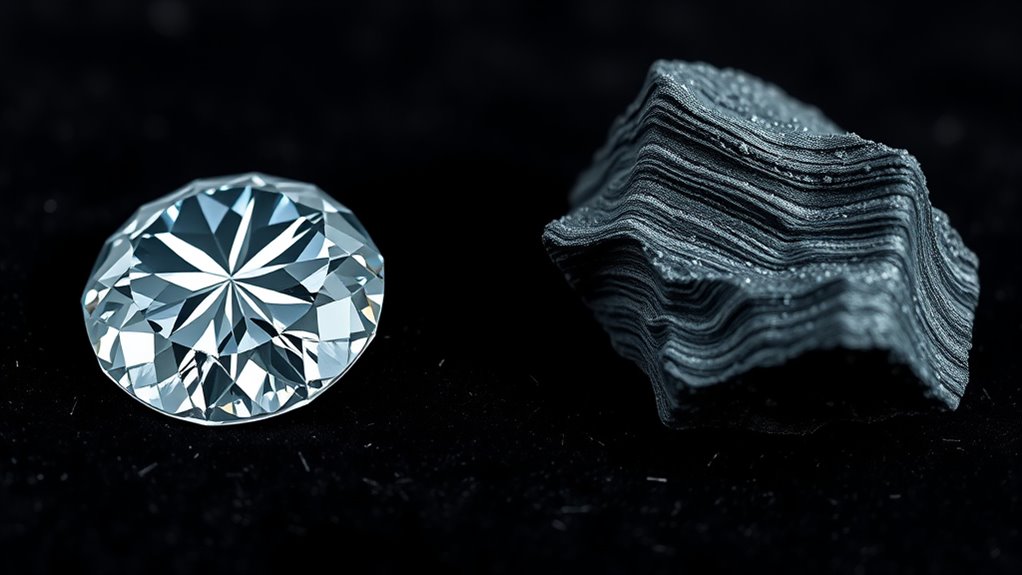
The structural differences between diamond and graphite lead to striking contrasts in their physical properties. Diamond is incredibly hard, rating 10 on the Mohs scale, thanks to its strong covalent bonds in a 3D network. In contrast, graphite is very soft, with a hardness of 1 to 2, because its layers are held together by weak van der Waals forces, allowing them to slide easily. Diamond has a higher density and appears transparent and colorless, while graphite is opaque, black, and dark grey. Graphite conducts electricity through delocalized electrons within its layers, whereas diamond is an insulator. Both withstand high temperatures, but diamond’s high thermal conductivity makes it ideal for heat management. Graphite’s layered structure gives it a slippery feel and makes it useful as a lubricant and in pencils. Additionally, understanding these allotropes highlights the importance of structural differences in determining their unique physical properties.
Chemical Reactivity and Behavior of Carbon Variants
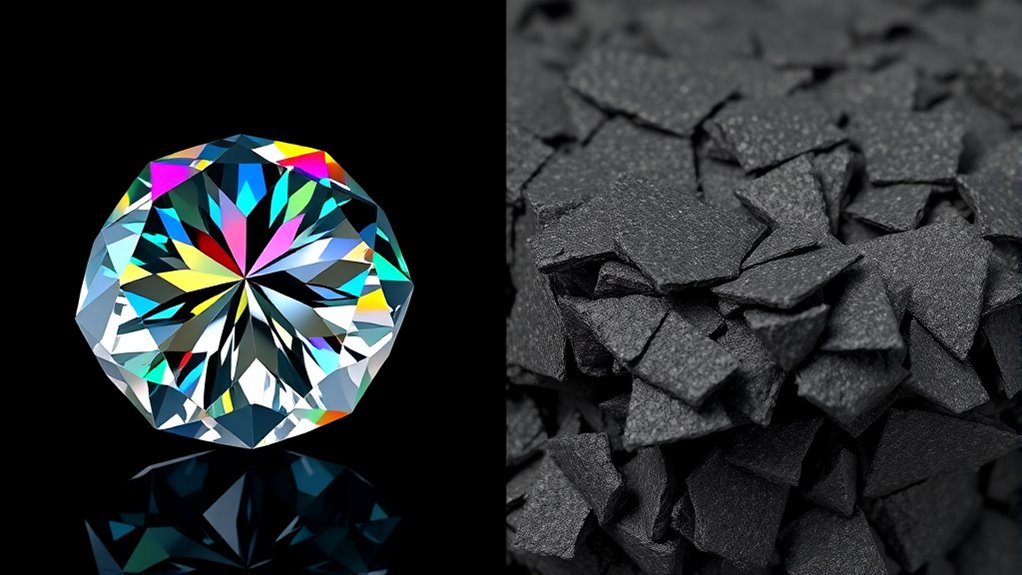
Have you ever wondered why some forms of carbon react more readily than others? It all comes down to their atomic structure and bonding.
Graphite’s layers of sp² hybridized carbons with delocalized π-electrons make it reactive, especially with oxidizers like nitric acid, despite being thermodynamically stable.
In contrast, diamond’s sp³ hybridized, tetrahedral network creates strong localized bonds, making it chemically inert at room temperature.
Fullerenes and nanotubes, also sp² hybridized, have curved or cylindrical surfaces that increase surface strain, boosting their reactivity and functionalization potential.
Atomic carbon, with unpaired electrons, is highly reactive and exists as a transient intermediate.
How Allotropes Influence Material Applications
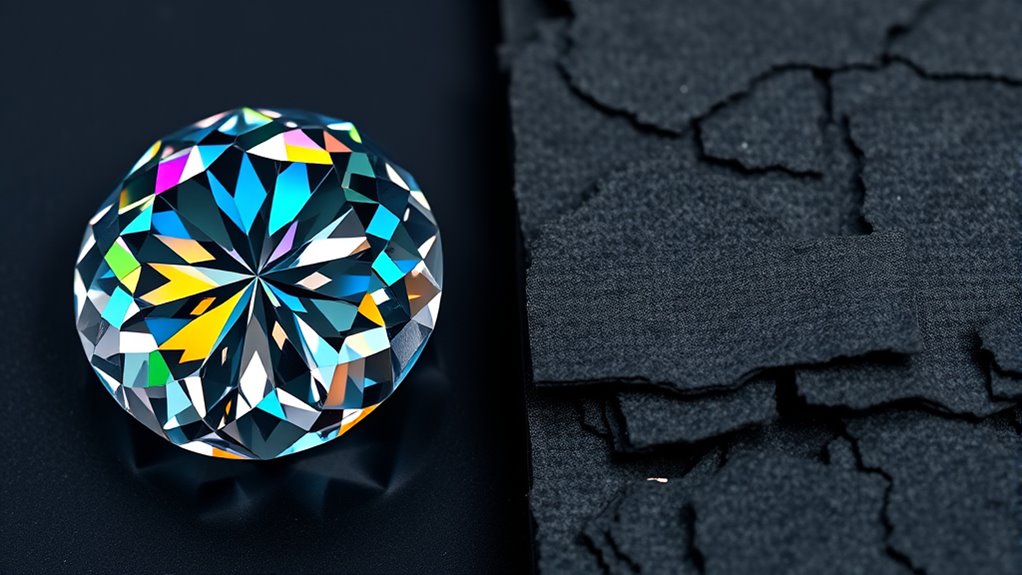
Different allotropes of carbon excel in various material applications because their unique structures and properties enable specialized functions. For example, graphene and carbon nanotubes are key in nanotechnology, thanks to their exceptional electrical and mechanical traits.
Graphite’s conductivity makes it ideal for electronic components and energy storage, like batteries. Diamond’s hardness and chemical stability suit cutting tools and jewelry. Graphite’s smooth texture reduces friction in lubricants.
Fullerenes, with their distinctive chemistry, are under research for medical uses and electronic components. These structural differences directly impact their strengths, conductivities, and stability, shaping their industrial roles. Additionally, the molecular structure of each allotrope influences how they interact with other materials, further expanding their potential applications.
Whether in aerospace, construction, or healthcare, the versatile properties of carbon allotropes allow for innovative, efficient, and specialized applications across many fields.
The Formation Conditions of Carbon Allotropes
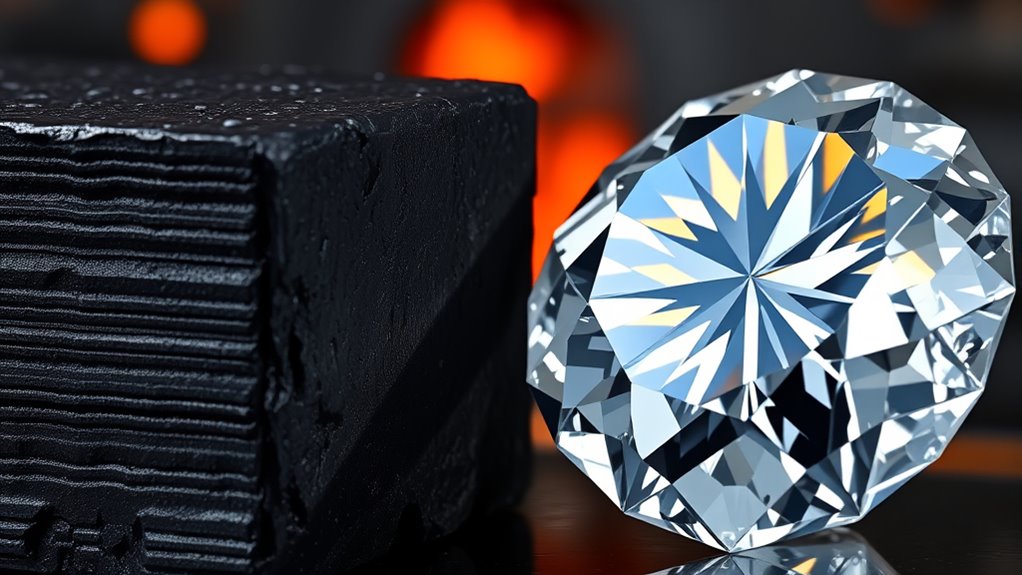
Carbon allotropes form through specific bonding arrangements that depend heavily on the environmental conditions during their creation. The way carbon atoms bond—mainly covalently—determines the structure and properties of each allotrope. High pressure and temperature conditions play a vital role; for example, diamond forms under intense pressure, creating a strong 3D network of bonds, while graphite develops in more moderate environments, with layers held together weakly. Synthetic methods, like chemical vapor deposition, can produce fullerenes and nanotubes by manipulating conditions such as vaporization and electric currents. Environmental factors like pressure and temperature influence whether carbon assembles into soft, layered graphite or the rigid, crystalline structure of diamond. These conditions ultimately guide the formation and stability of each carbon allotrope, with bonding arrangements playing a critical role in defining their unique properties.
Stability and Transformation of Allotropic Forms
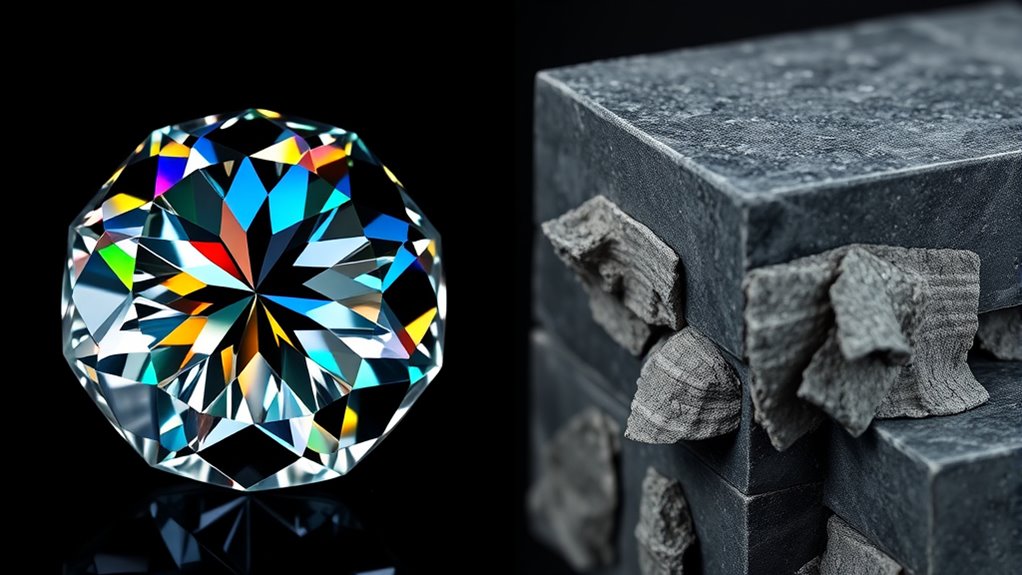
Understanding the stability of allotropes requires examining how resistant they’re to changing their structure or transforming into other forms. Stable allotropes sit in deep potential wells on the energy landscape, with high barriers preventing easy change. Unique and wicked planters demonstrate how material choices influence stability and aesthetics in practical applications. For example, graphite is more stable under normal conditions, while diamond becomes stable at high pressures and temperatures. High transformation barriers mean specific conditions, such as heat, pressure, or catalysts, are needed to induce changes. Usually, transformations favor the thermodynamically stable form, like graphite converting to diamond under pressure. Structural stability varies: graphite resists burning, whereas diamond’s rigidity makes it extremely hard. Less stable forms, like penta-graphene, tend to quickly transform into more stable structures, emphasizing how external conditions influence an allotrope’s stability and potential for transformation.
Industrial Production of Diamond and Graphite
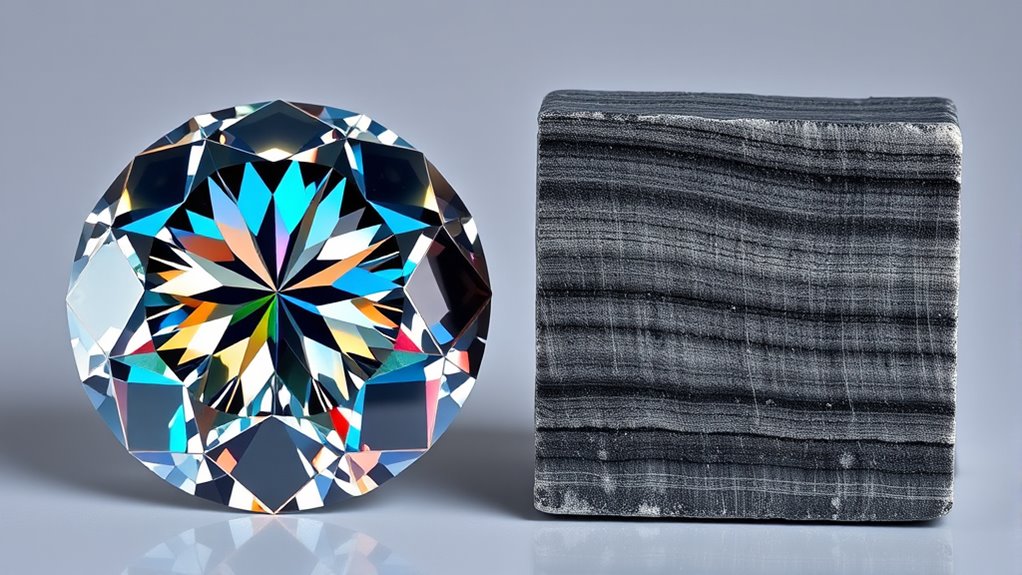
Industrial production of diamonds and graphite involves specialized methods that replicate natural processes or utilize advanced technology.
For diamonds, the HPHT method uses high pressure (over 5 GPa) and high temperatures (around 1,500°C) to imitate natural formation. The CVD process deposits carbon plasma onto substrates, producing high-quality gem diamonds with precision. Detonation nanodiamonds are created through controlled explosions, yielding nano-sized particles for specialized applications.
Post-synthesis, diamonds are cut, polished, and processed for uses like cutting tools and electronics. High-pressure techniques are crucial in synthesizing diamonds with specific qualities.
Graphite production relies on the Acheson process, where petroleum coke mixed with coal tar pitch is heated in electric furnaces at 2,500–3,000°C, followed by purification. These methods highlight how high energy input and specific conditions are essential to produce each allotrope efficiently.
The Role of Allotropes in Modern Technology
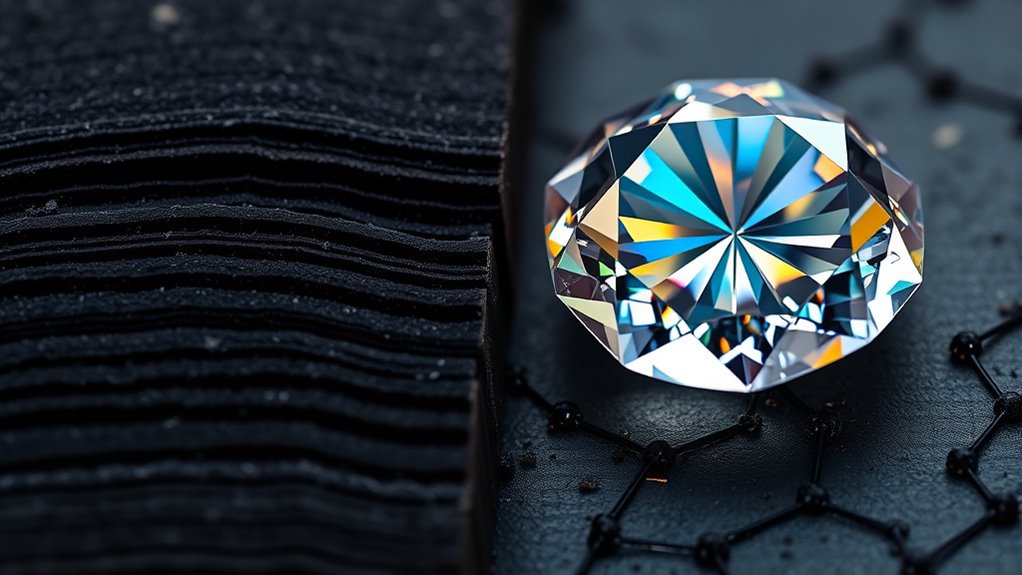
Allotropes like graphene, diamond, and carbon nanotubes have revolutionized modern technology by offering unique combinations of electrical, thermal, and mechanical properties. You benefit from graphene’s exceptional electrical conductivity in touch screens and flexible displays, while diamond’s thermal conductivity and insulating qualities enable high-power electronics. A variety of vetted options are available for integrating these materials into different applications. Carbon nanotubes provide high strength and conductivity, ideal for nanoelectronics and sensors. These materials boost flexible electronics’ durability and performance. In energy storage, graphene’s large surface area enhances batteries and supercapacitors, and nanotubes improve charge transport. Allotropes also strengthen composites used in aerospace and automotive industries. Their versatility extends to biomedical applications, with diamond’s biocompatibility and graphene’s functionalization enabling implants, biosensors, and drug delivery systems. These advancements underscore how carbon allotropes drive innovation across multiple fields.
Natural Occurrence and Geological Context of Allotropes
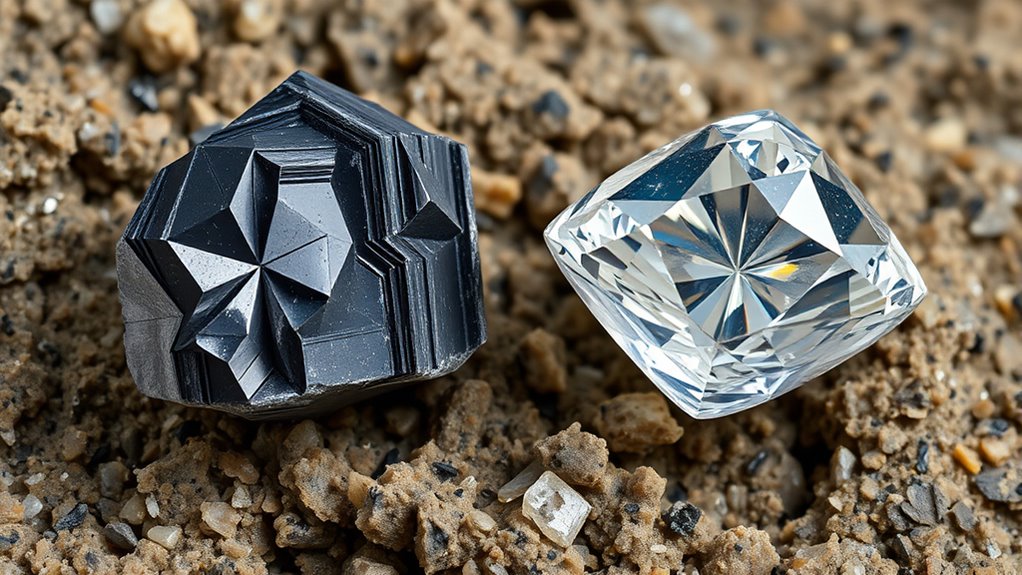
Diamonds and graphite, the two most well-known natural carbon allotropes, form under vastly different geological conditions that influence their distribution and occurrence. Diamonds develop deep within the Earth’s mantle at depths of 140–190 km, where high pressure and temperature enable their formation. High-pressure environments are essential for diamond genesis, making their natural occurrence quite rare. Volcanic eruptions rapidly transport these gemstones to the surface via kimberlite and lamproite pipes. In contrast, graphite forms through metamorphism of carbon-rich sediments in rocks like schist and gneiss, often in regions with intense geological activity, such as ancient mountain belts. While diamonds are rare, graphite is more abundant due to its stability at Earth’s surface conditions. Natural nanostructures like fullerenes have also been found in fossil material, but their occurrences are much less common.
Frequently Asked Questions
Can All Elements Have Multiple Allotropes?
You might wonder if all elements can have multiple allotropes. In reality, not every element does. Only some, like carbon, oxygen, and sulfur, show different structural forms because of their bonding capabilities.
Many elements, especially metals, tend to have a single stable form. Factors like atomic structure and bonding preferences limit the existence of multiple allotropes.
How Do Temperature and Pressure Influence Allotrope Stability?
Temperature and pressure play key roles in determining which allotrope is most stable.
You’ll find that increasing temperature can cause phase shifts or crystal structure changes, while high pressure often favors denser forms, like diamond from graphite.
These conditions shift the stability balance, causing transformations or maintaining specific allotropes within certain ranges.
Are There Synthetic Allotropes Not Found Naturally?
You might be surprised to learn that many synthetic allotropes don’t exist naturally. For example, carbon nanotubes and graphene were both created in labs after their natural counterparts weren’t found.
In fact, graphene’s discovery in 2004 revolutionized materials science, showing how humans can engineer structures with properties impossible in nature.
These synthetic allotropes open exciting possibilities for electronics, energy, and medicine, far beyond what natural forms can offer.
How Does Allotropy Affect the Electrical Conductivity of Elements?
Allotropy influences an element’s electrical conductivity by changing its atomic arrangement and bonding.
When atoms form structures with delocalized electrons, like graphite, they conduct electricity well.
But if the atoms create strong covalent bonds with localized electrons, as in diamond, the material acts as an insulator.
Can Allotropes Transform Into Each Other Under Normal Conditions?
You might think all transformations happen easily, but in reality, most allotropes are quite shy about changing under normal conditions. For example, diamond and graphite, both forms of carbon, stay as they’re unless you apply extreme pressure or heat.
Typically, these changes require special environments, so under everyday circumstances, allotropes remain steadfast in their forms, making their transformations a rare and fascinating event.
Conclusion
Understanding allotropes reveals how the same element can have vastly different properties. Did you know that diamonds are the hardest natural substance, while graphite is one of the softest? This striking difference highlights how structure defines function. By exploring these forms, you see their importance in technology and industry. Next time you see a diamond or pencil lead, remember they’re both carbon, yet worlds apart in their amazing properties.