Carbon Capture & Utilisation (CCUS) involves capturing CO₂ from industrial sources or power plants, transporting it safely, and either injecting it underground for long-term storage or transforming it into useful products like fuels, chemicals, or construction materials. Technologies include chemical absorption, membrane separation, and mineralization. Safety, environmental impacts, policy incentives, and recent innovations play key roles. If you keep exploring, you’ll discover how these systems are evolving to fight climate change effectively.
Key Takeaways
- CCUS captures CO2 from industrial sources using technologies like chemical absorption, membrane separation, and looping cycles for high efficiency.
- CO2 transport primarily uses pipelines, with alternatives like trucks and ships, to move captured carbon to storage or utilization sites.
- Geological storage involves injecting CO2 into depleted oil fields or saline aquifers, requiring monitoring and safety measures to prevent leaks.
- CO2 utilization converts captured carbon into synthetic fuels, chemicals, mineralized materials, or biomass, supporting sustainable industries.
- Challenges include high costs, regulatory hurdles, infrastructure development, and public safety concerns, while future trends focus on integration with renewables and modular systems.
Understanding the Fundamentals of CCUS
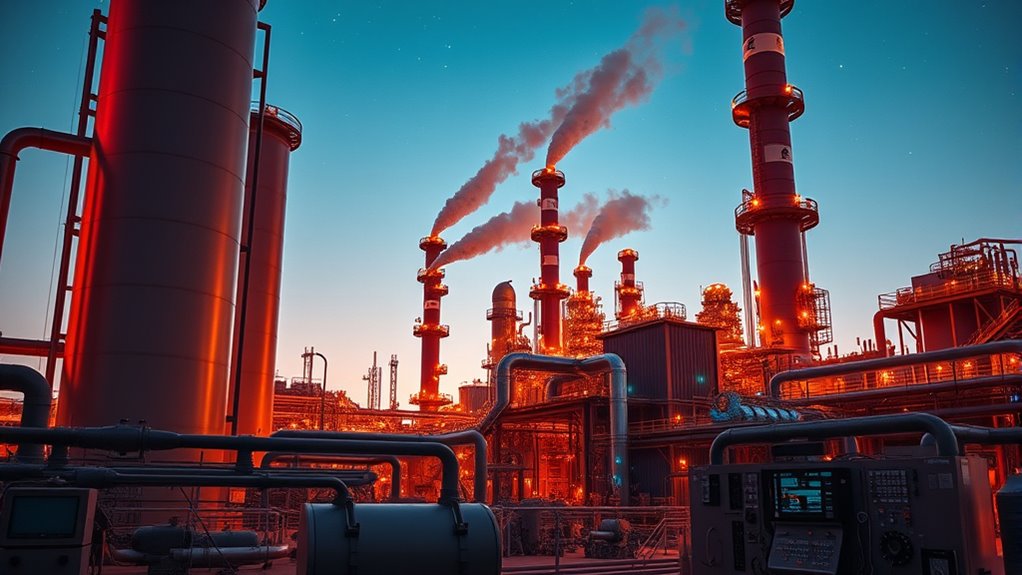
Understanding the fundamentals of CCUS starts with recognizing how it captures carbon dioxide emissions before they reach the atmosphere. The process involves collecting CO2 from industrial sources or power plants, preventing it from contributing to climate change. This step is crucial for reducing the amount of greenhouse gases released into the atmosphere. Once captured, the CO2 can be used in various ways, such as in construction materials or enhanced oil recovery, or stored underground in geological formations designed to trap it permanently. Effective sequestration requires the CO2 to stay isolated for centuries, making geologic storage the most mature method. Currently, global projects store millions of tonnes of CO2 annually, but to meet international climate goals, scaling up to billions of tonnes per year is necessary. This approach helps reduce greenhouse gases and supports global efforts to combat climate change. Additionally, ongoing research and development are vital to improving the efficiency and safety of carbon sequestration methods.
Key Technologies and Capture Methods
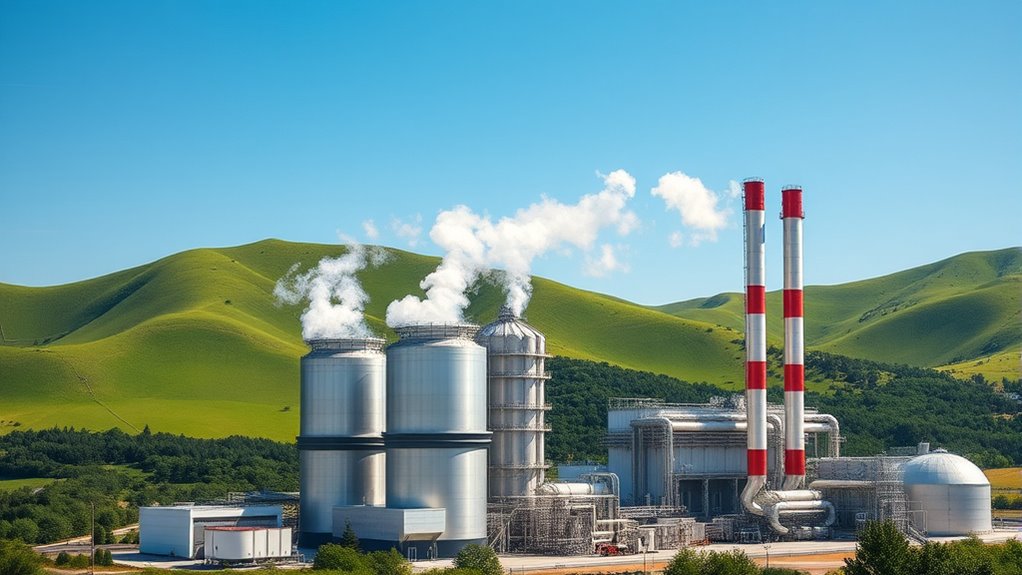
Various technologies are employed to capture CO2 emissions effectively, each suited to different industrial processes and gas compositions. Chemical absorption is the most mature, using solvents like amines to bond with CO2, achieving around 90% efficiency and up to 99% under *ideal* conditions. It’s ideal for post-combustion capture but requires significant energy, increasing costs. Juice cleansing can sometimes cause temporary side effects like fatigue and headaches, highlighting the importance of health considerations in detox practices. Physical separation techniques, such as cryogenic separation and pressure swing adsorption, work well with high CO2 partial pressures, like natural gas processing, and consume less energy but are less effective at low CO2 levels. Membrane-based capture is emerging, offering modular, energy-efficient solutions, though challenges remain in durability and purity. Looping cycles, including chemical and calcium looping, show promise for high capture rates with lower energy penalties, currently advancing through research and pilot projects.
Transport and Storage of Captured CO₂
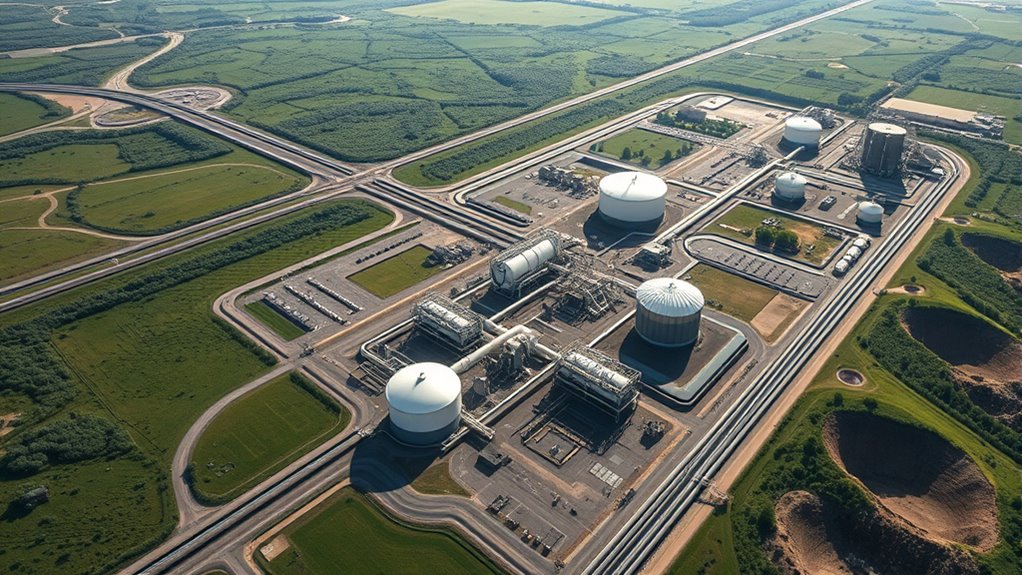
Transporting captured CO₂ efficiently and safely is essential for effective carbon management. Pipelines are the most common method, using pressure differences to move gases quickly and reliably. Compressing and liquefying CO₂ reduces its volume, often into a supercritical fluid, which combines high density with low viscosity, making transport more efficient. Trucks and ships are alternatives but less efficient, suitable for shorter distances or specific locations. Safety is crucial—pipelines can leak, so monitoring and using compatible materials prevent accidents. High-pressure conditions minimize leakage risks. Strict regulations and emergency plans further ensure safety. Proper transport is vital for delivering CO₂ to storage sites like depleted oil fields or saline aquifers, supporting long-term carbon removal efforts. Incorporating regulatory compliance measures ensures adherence to safety standards and minimizes environmental impact during transport.
Innovative Uses and Applications of CO₂
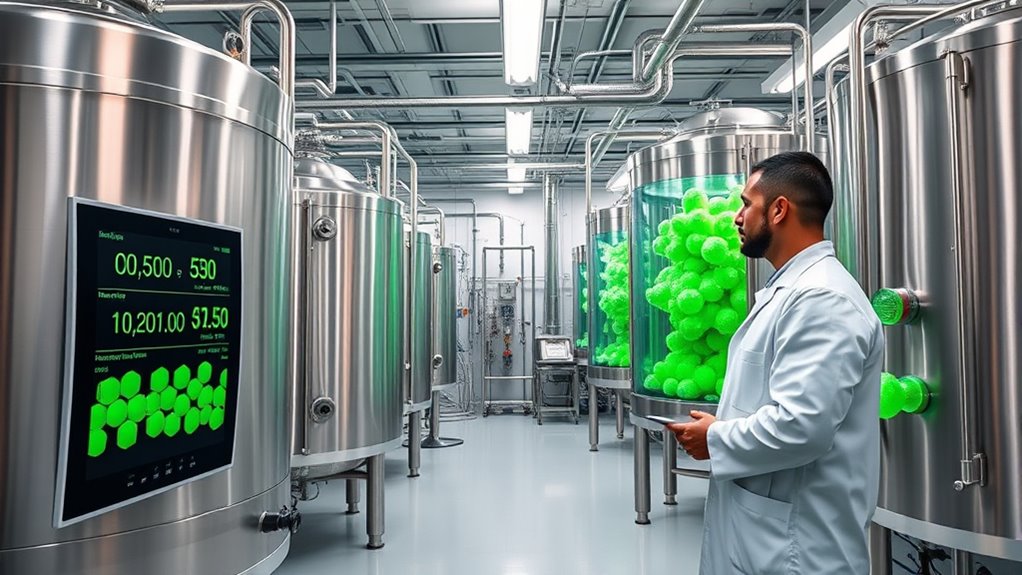
Innovative uses of captured CO₂ are transforming it from a waste product into a valuable resource across multiple industries. You can convert CO₂ into synthetic fuels like methanol and aviation fuels, offering sustainable alternatives to fossil fuels.
Chemical production also benefits, producing formic acid and ethylene—key precursors for plastics and chemicals—using electrochemical conversion powered by renewable energy.
Electrochemical conversion of CO₂ creates essential chemicals like formic acid and ethylene with renewable energy.
In construction, CO₂ mineralization creates stable carbonate materials for concrete and aggregates, permanently locking carbon.
Agriculture harnesses CO₂ for fertilizers and greenhouse enrichment, boosting crop yields sustainably.
Biotechnology leverages microbes and algae to produce biofuels, bioplastics, and chemicals from CO₂, advancing renewable product development.
Additionally, direct air capture provides a clean feedstock, supporting the circular carbon economy and reducing overall emissions.
Carbon capture and utilization technologies are advancing rapidly, providing scalable solutions for global emissions reduction.
These innovations are shaping a greener, more sustainable future.
Overcoming Challenges in CCUS Deployment
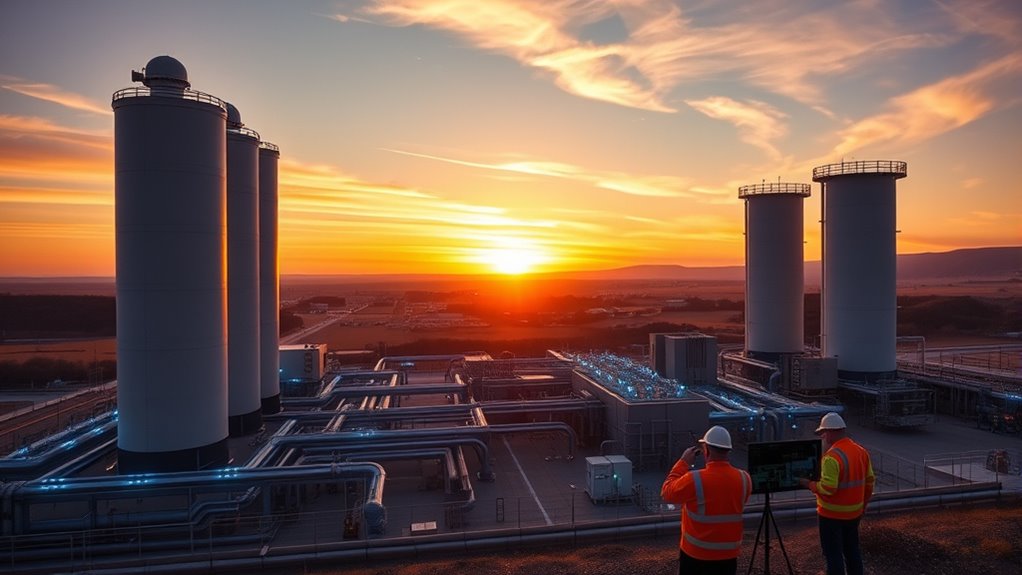
While the potential of CCUS technologies to reduce emissions is promising, deploying these systems faces significant hurdles. High upfront costs for capturing, transporting, and storing CO₂ make projects financially risky. The energy needed for capture and compression adds operational expenses, while unclear carbon pricing and limited incentives hinder investment confidence. Effective organization can help streamline project management and optimize resource allocation, making deployment more feasible. Regulatory frameworks are often incomplete or inconsistent, causing delays and uncertainty. Developing suitable storage sites and building transportation infrastructure require substantial coordination and capital, facing long timelines and engineering challenges. Stakeholder alignment is difficult, with risks of stranded assets and market instability discouraging early investments. Public concerns about safety and environmental impacts further complicate deployment. Overcoming these barriers demands clearer policies, streamlined regulations, and stronger market mechanisms to foster trust and investment.
The Role of CCUS in Emission Reduction Strategies
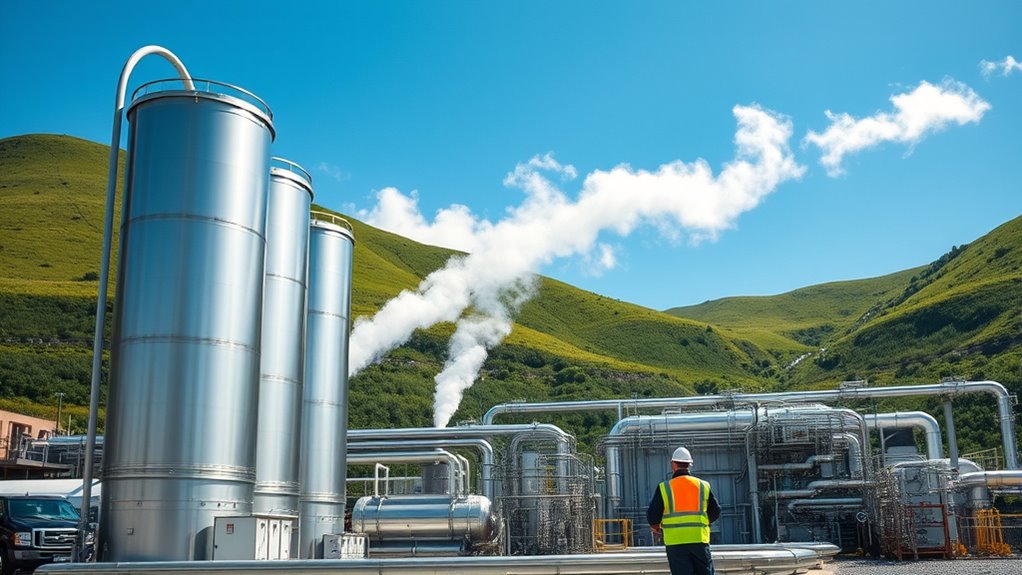
CCUS plays a pivotal role in reducing emissions across various sectors, especially where decarbonization options are limited. You can retrofit existing fossil-fuel power plants and industrial facilities to cut emissions without decommissioning them.
CCUS enables emission reductions in existing fossil-fuel plants and industries without needing decommissioning.
It targets hard-to-abate industries like cement, steel, and chemicals, where alternatives are scarce. CCUS also supports clean hydrogen production by capturing CO2 during fuel processing, enabling low-carbon fuels.
Additionally, it facilitates biomass energy with CCS (BECCS), achieving net-negative emissions by removing CO2 from the atmosphere. The technology adds system flexibility, balancing renewable energy and providing dispatchable power.
As an essential tool, CCUS helps meet net-zero targets, avoids “carbon lock-in,” and guarantees a stable, resilient energy system while addressing emissions in sectors that are difficult to decarbonize.
Emerging Trends and Future Innovations
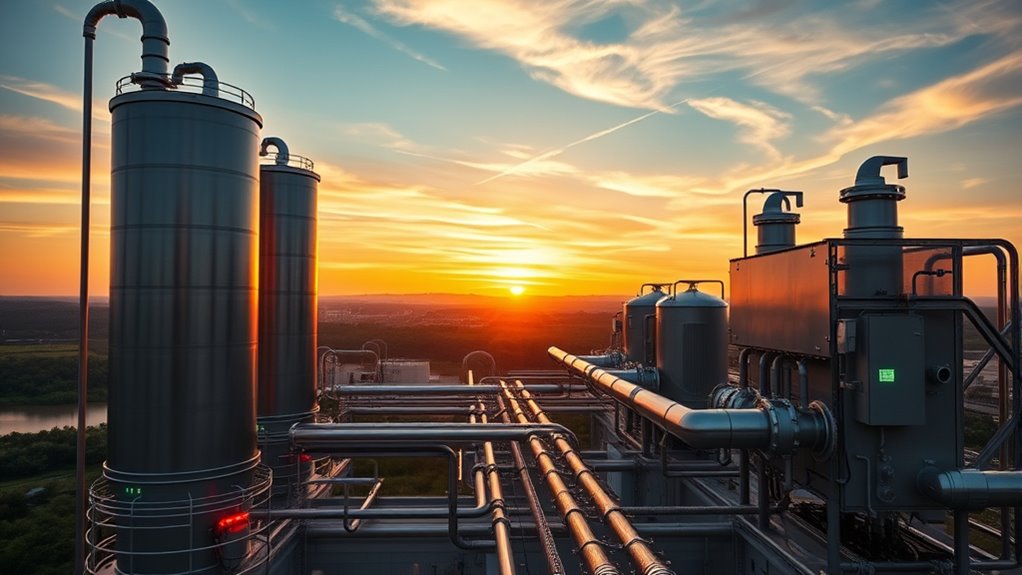
Emerging trends in CCUS highlight rapid market growth, driven by increasing investments and technological advancements. The global market is projected to grow from $3.4 billion in 2024 to $9.6 billion by 2029, with a capture capacity expected to reach 2.5 gigatonnes annually by 2045.
As of 2023, announced capacity has increased markedly, especially in hydrogen, power, and industrial sectors.
Technologies are becoming more modular and scalable, reducing costs and boosting efficiency. Innovations in transport and storage infrastructure, along with the integration of renewable energy, are enhancing system safety and performance.
Policy developments like the EU’s CBAM and corporate reporting requirements are further accelerating adoption.
Leading companies are pushing boundaries, fostering a vibrant ecosystem of innovation that aims to transform CCUS into a crucial tool for decarbonization. Advancements in technology are making these systems more effective and accessible worldwide.
Environmental and Safety Considerations
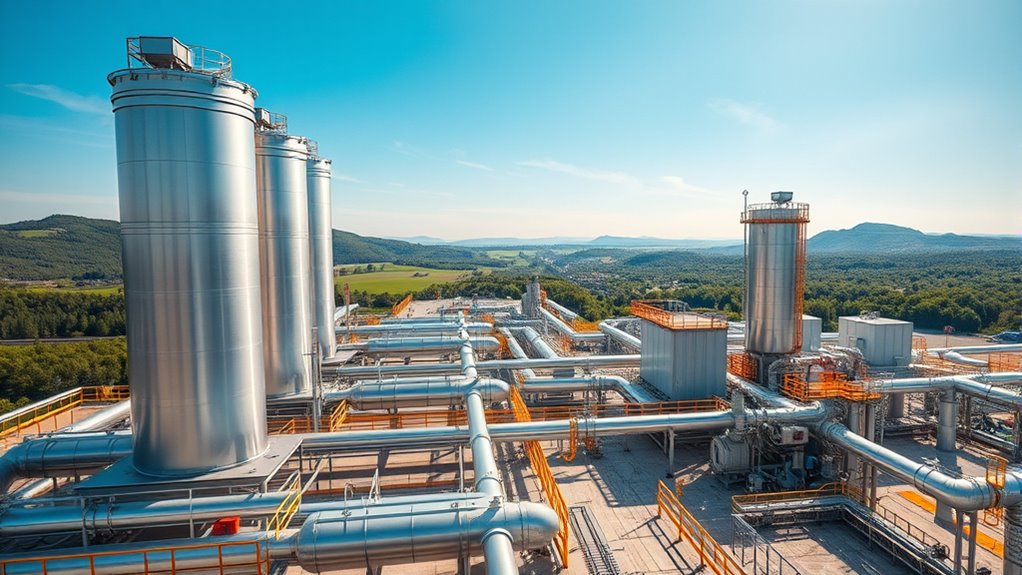
As the market for carbon capture and utilization expands rapidly, addressing environmental and safety concerns becomes increasingly important. You need to be aware of risks like CO2 leaks or pipeline ruptures, which can cause asphyxiation hazards.
Marginalized communities, such as those in Louisiana’s “Cancer Alley,” face disproportionate dangers from CCS infrastructure. Underground storage poses threats like groundwater contamination and induced seismic activity.
While CCS reduces emissions, it doesn’t eliminate industrial pollution, and community opposition often favors renewable energy over CCS projects.
Infrastructure impacts include habitat fragmentation, land disputes, and energy-intensive operations that increase lifecycle emissions.
Long-term monitoring is essential to prevent leakage, but legal and safety frameworks remain unclear. Managing these risks requires careful planning to protect public health and the environment.
Furthermore, the Private Placement Equity Markets landscape influences how projects are financed and implemented, underscoring the importance of secure investment strategies that also consider environmental safeguards.
Policy Frameworks and Economic Incentives
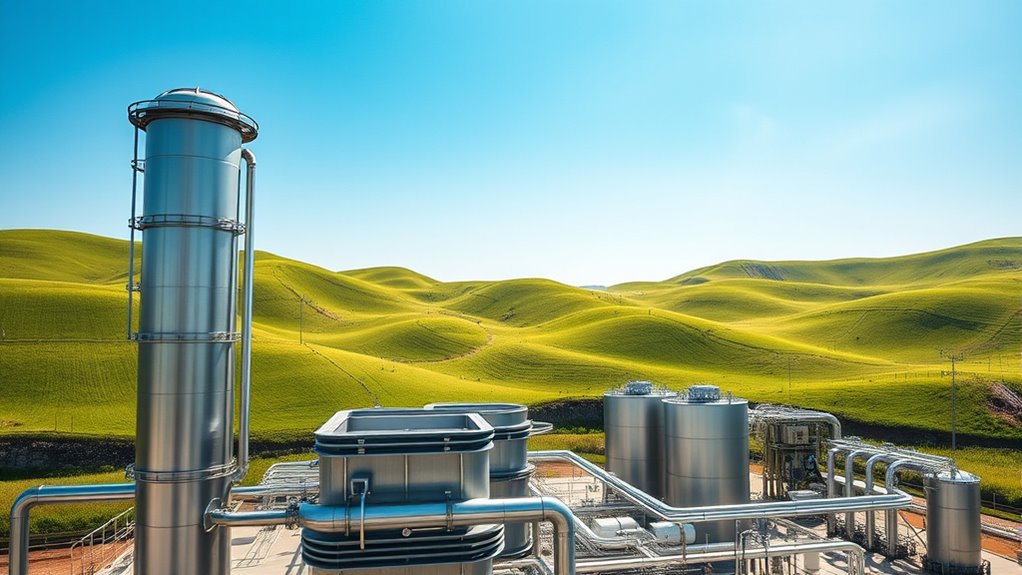
Policy frameworks and economic incentives play a crucial role in driving the adoption and scaling of carbon capture and utilization (CCU) technologies. The U.S.’s 2025 Federal Policy Blueprint offers a clear national strategy, while laws like the 2022 Section 45Q tax credit enhancements provide strong financial support for CCU projects.
The Infrastructure Investment and Jobs Act (2021) allocated funding specifically for demonstration and deployment. Streamlining permitting through regulatory timelines and a designated “one-stop-shop” agency can reduce delays. At the state level, tailored regulations address regional needs and support federal incentives.
Economically, tools like the 45Q tax credit, Carbon Contracts for Difference, and investment tax credits encourage private investments. Grants, public-private partnerships, and international models further accelerate CCU technology deployment. Additionally, incorporating environmental considerations such as sustainable practices and ecosystem protection can enhance long-term project viability and community support.
Case Studies and Real-World Implementations
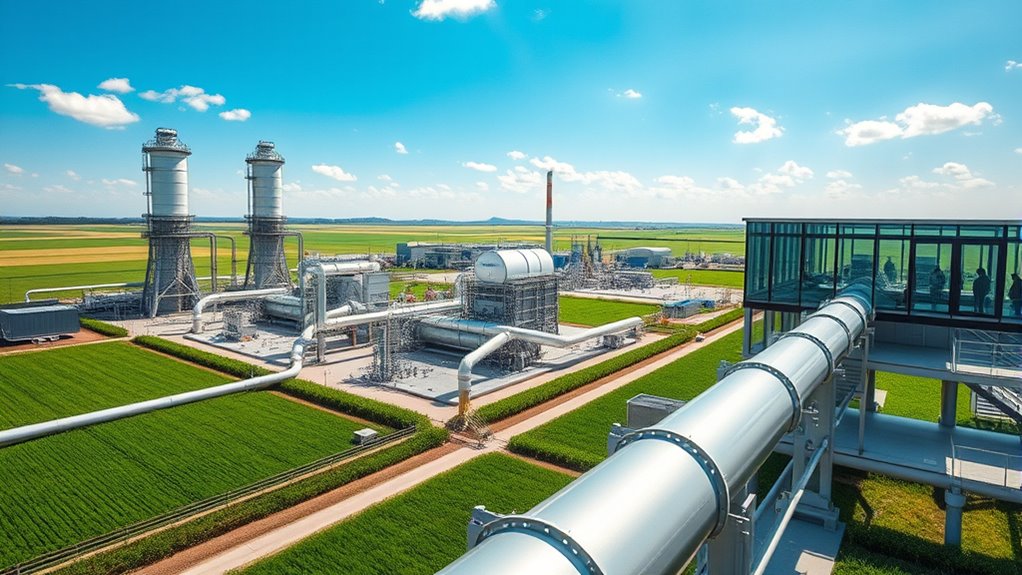
Real-world implementations of carbon capture and utilization (CCU) demonstrate how these technologies perform outside of lab settings. The Petra Nova project in the USA, started in 2015, captured about 1.4 million tons of CO2 annually from a coal plant, mainly for enhanced oil recovery. Despite technical success, high costs limited economic viability.
Real-world CCU projects like Petra Nova show technical success but face economic challenges.
The Midwest Regional Carbon Sequestration Partnership has safely stored over 2 million tons of CO2 in geologic formations, while producing oil through EOR, showcasing large-scale regional potential.
CarbonQuest’s modular units capture up to 300,000 tons per year, mineralizing CO2 into rock at local cement plants and targeting nearly 80,000 tons annually.
Industrial facilities across sectors retrofit to capture and utilize CO2, demonstrating versatility, while power plants equipped with CCS units balance power needs with emission reductions.
Recent advancements in self-balancing scooters for kids highlight the importance of safety features and suitability for supervised use in various environments, which can also inform safety considerations in CCU technology deployment.
Frequently Asked Questions
How Does CCUS Impact Overall Energy Consumption?
You might notice that CCUS increases overall energy consumption because the capture process is energy-intensive, requiring 15-25% of a power plant’s output.
This extra energy demand means less is available for electricity generation, potentially lowering efficiency.
However, by using renewable energy sources to power capture systems, you can balance this increased consumption and reduce long-term emissions, supporting a cleaner energy progression.
What Are the Long-Term Risks of Underground Co₂ Storage?
You’re asking about the long-term risks of underground CO₂ storage. Over time, geological instability, such as fractures or earthquakes, could cause leaks.
Infrastructure issues like well failures or abandoned wells might also lead to environmental hazards.
Additionally, unpredictable chemical reactions and pressure changes could jeopardize storage integrity.
While monitoring technologies improve, uncertainties remain, and long-term risks require careful management to prevent groundwater contamination and ensure environmental safety.
Can CCUS Be Integrated With Renewable Energy Systems?
Yes, you can integrate CCUS with renewable energy systems to improve reliability and reduce emissions.
By powering carbon capture processes with renewable electricity, you lower the carbon footprint of capture operations. This combination also helps stabilize renewable energy supply fluctuations, making your low-carbon energy system more resilient.
Plus, integrating these technologies can lower costs, enable new pathways for decarbonization, and enhance overall system efficiency and sustainability.
What Are the Main Barriers to Global CCUS Adoption?
Think of the global push for CCUS like trying to steer a ship through stormy waters—you face many obstacles. You’ll encounter hefty upfront costs, limited financial incentives, and patchy policies that slow progress.
Technological gaps and public skepticism act like turbulent waves, making steady advancement difficult. Without consistent international rules and stronger societal support, your efforts to adopt CCUS worldwide remain adrift in uncertainty.
How Does CCUS Influence the Economics of Fossil Fuel Industries?
You see that CCUS influences fossil fuel economics by extending plant lifespans and creating new revenue streams like enhanced oil recovery, but it also raises costs considerably.
You’ll notice high upfront investments and increased operational expenses, often needing government support or subsidies to stay viable.
While it helps fossil fuel industries meet regulations and maintain competitiveness, it also risks stranded assets and profit margins squeezed without policy incentives.
Conclusion
As you explore the future of CCUS, imagine a world where captured CO₂ transforms into new resources, reshaping industries and safeguarding our planet. The path ahead is filled with breakthroughs and challenges alike, each step revealing more about what’s possible. Will innovation unseal a sustainable tomorrow? The journey continues, and the choices you make now could determine whether this technology becomes a beacon of hope or a missed opportunity. The future of carbon capture hangs in the balance—are you ready to shape it?