Understanding the chemistry behind radiation therapy and radiopharmaceuticals helps you see how they target cancer precisely while sparing healthy tissue. Researchers develop molecules that attach radioactive elements to tumor-specific receptors, using chelators and engineered linkers for stability. Advances in chemistry improve radionuclide design, delivery systems like nanoparticles, and immune activation. By exploring these chemical strategies, you’ll discover how they enhance treatment efficacy and pave the way for personalized cancer therapies that continue to evolve.
Key Takeaways
- The chemistry of radiopharmaceuticals involves designing stable linkers, targeting molecules, and selecting appropriate radionuclides for precise tumor delivery.
- Targeted radiopharmaceuticals utilize receptor-specific molecules like antibodies or peptides to improve tumor specificity and minimize healthy tissue damage.
- Chelating agents securely bind radionuclides, ensuring stability and proper positioning within biological molecules for effective therapy.
- Advanced delivery systems like liposomes and nanoparticles facilitate deep tumor penetration and controlled release of radioactive payloads.
- Innovations in chemical strategies aim to enhance targeting accuracy, reduce side effects, and support personalized cancer treatment approaches.
The Fundamentals of Radiation-Induced DNA Damage and Repair Mechanisms
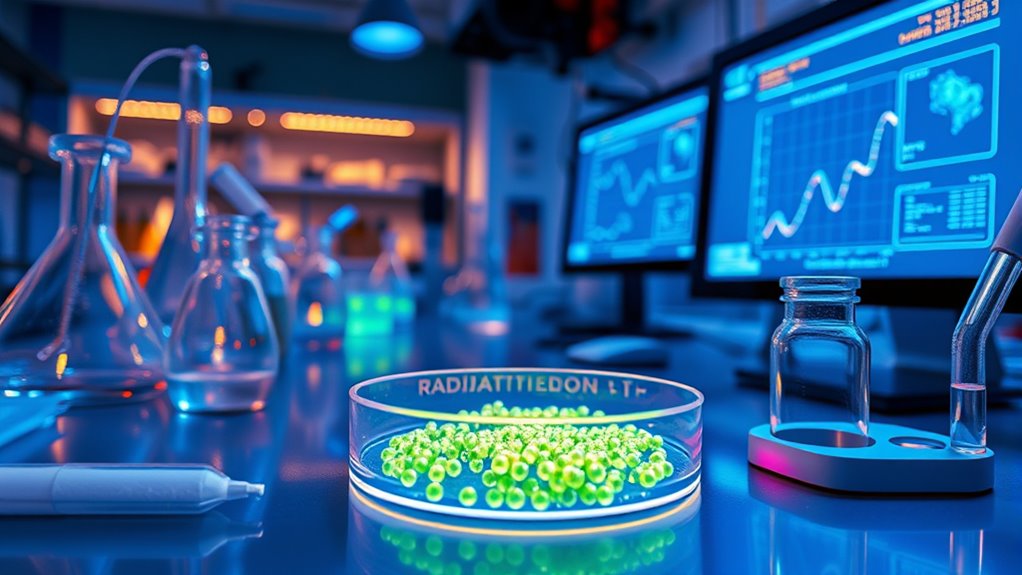
How does radiation damage DNA, and what repair mechanisms do cells use to counteract this? When radiation passes through cells, it causes various DNA lesions. These include base damage, such as oxidation or alkylation, and strand breaks—single-strand breaks (SSBs) and the more lethal double-strand breaks (DSBs).
High-LET radiation produces complex, clustered damage, making repair more challenging. Your cells activate DNA Damage Response (DDR) pathways, like ATM/ATR kinases, to detect DSBs and initiate repair.
Single-strand breaks and damaged bases are fixed via base excision repair (BER), while DSBs are repaired through non-homologous end joining (NHEJ) or homologous recombination (HR), depending on the cell cycle phase.
These mechanisms maintain genomic stability but can be overwhelmed by intense or complex damage. Understanding these repair pathways is essential for developing more effective cancer treatments that exploit the cell’s repair vulnerabilities.
How Radiopharmaceuticals Target Tumors Through Chemistry
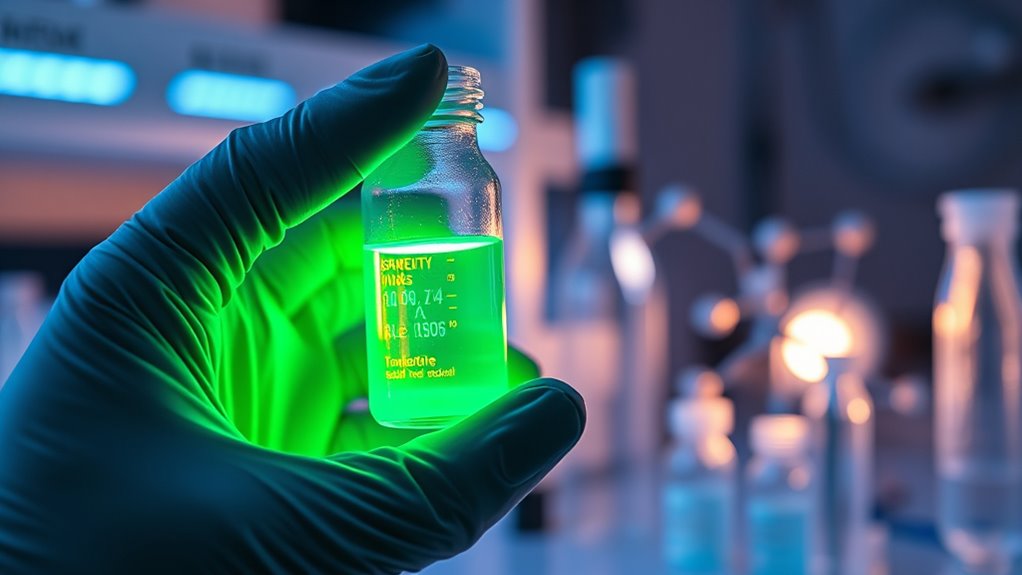
Radiopharmaceuticals are designed to deliver targeted radiation therapy directly to tumor cells by harnessing specific chemical interactions. They consist of three main parts: a radioactive molecule for therapy, a targeting molecule that recognizes cancer markers, and a linker that connects them. The targeting molecule binds selectively to tumor-specific receptors like HER2 or PSMA, ensuring precise delivery. Radionuclides mimic physiological substances or use covalent bonds to stay attached to tumor proteins, enhancing efficacy. The linker chemistry maintains stability and proper orientation, preventing premature release and reducing side effects. Different radionuclides, such as alpha or beta emitters, are chosen based on tumor size and location. The selection of radionuclides also depends on their half-life and energy emission, which influence their suitability for specific types of tumors. This strategic combination of chemistry enables radiopharmaceuticals to deliver radiation doses precisely where needed, maximizing tumor destruction while sparing healthy tissue.
The Role of Cell Cycle and Repair Pathways in Radiosensitivity
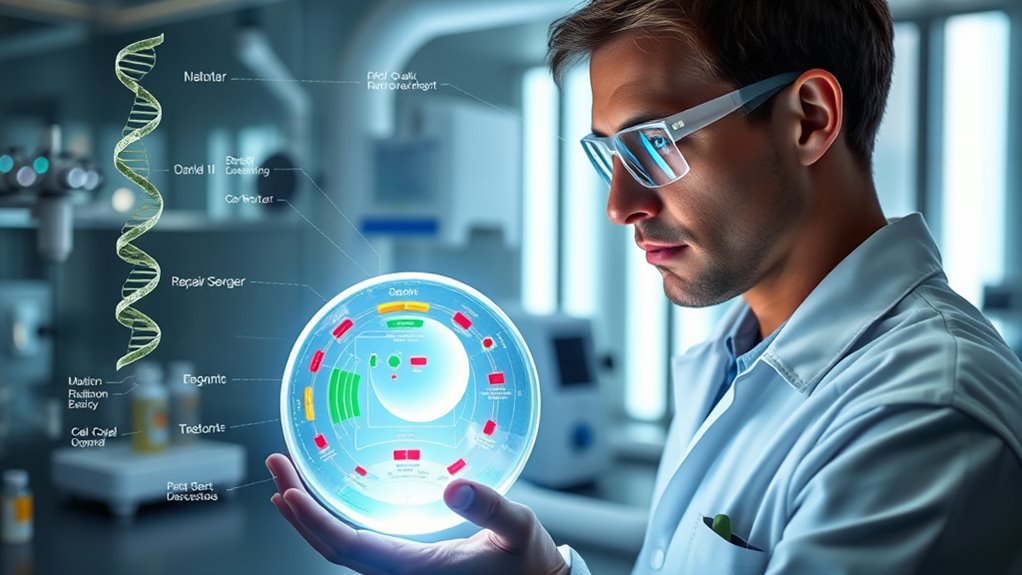
The cell cycle plays a critical role in determining a cell’s sensitivity to radiation, with certain phases being more vulnerable than others. You’ll find cells are most radiosensitive in the G2-M phase, less so in G1, and least sensitive during late S phase. Radiation often causes cell cycle arrest, trapping cells in G2-M and increasing their vulnerability. The p53 gene mediates checkpoints at G1-S and G2-M, influencing how cells respond to damage. Cancer cells, with disrupted cycle control, often resist radiation. DNA damage activates repair mechanisms like homologous recombination and nonhomologous end-joining, which can either fix damage or lead to cell death if repair fails. Efficient repair within 48 hours reduces radiosensitivity, but incomplete repair prolongs G2/M arrest, increasing cell death. The effectiveness of repair pathways also depends on the cell cycle stage, affecting overall radiation response. Additionally, the capacity for DNA repair can vary among different cell types, further impacting radiosensitivity.
Enhancing Treatment Efficacy With Chemically Engineered Radionuclides
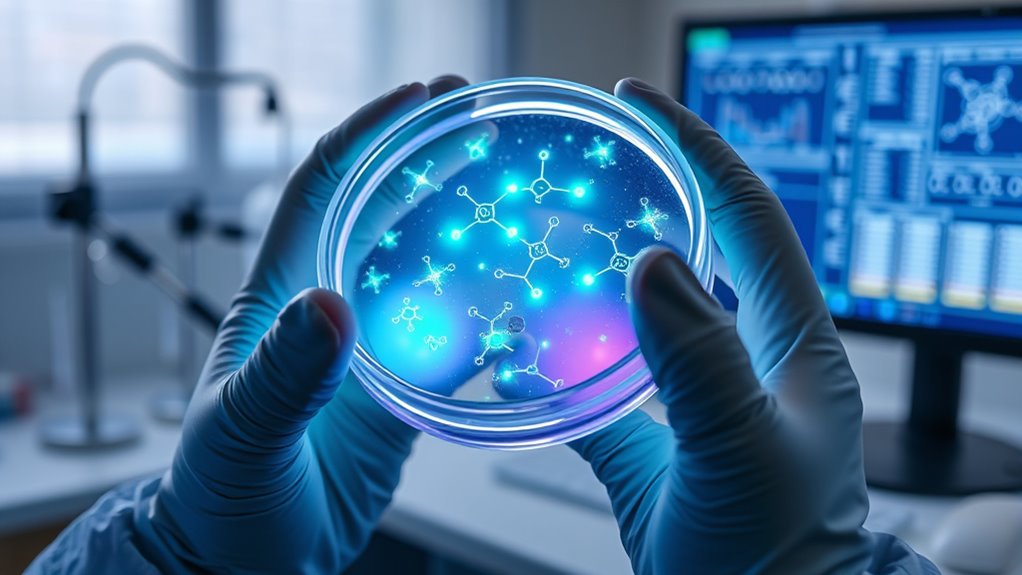
Advances in chemical engineering have markedly improved the precision and effectiveness of radionuclide-based therapies. You can now design radiopharmaceuticals that target tumor-specific receptors or antigens, boosting molecular-level accuracy. Targeting specific tumors Chelating ligands are engineered to securely hold radionuclides within biologically active molecules like antibodies or peptides, ensuring delivery directly to cancer cells. Conjugation techniques link alpha- or beta-emitting radionuclides to high-affinity tumor-targeting agents, maximizing localized radiation. Functionalization with tumor-homing peptides enhances receptor-mediated uptake, increasing therapeutic impact while protecting normal tissue. Additionally, contrast ratio molecular engineering reduces off-target effects by optimizing radionuclide stability, binding kinetics, and payload delivery within the biological environment. These innovations enable more precise, effective treatments, minimizing collateral damage and improving patient outcomes.
Immunological Effects of Radiation and the Chemistry Behind Immune Activation
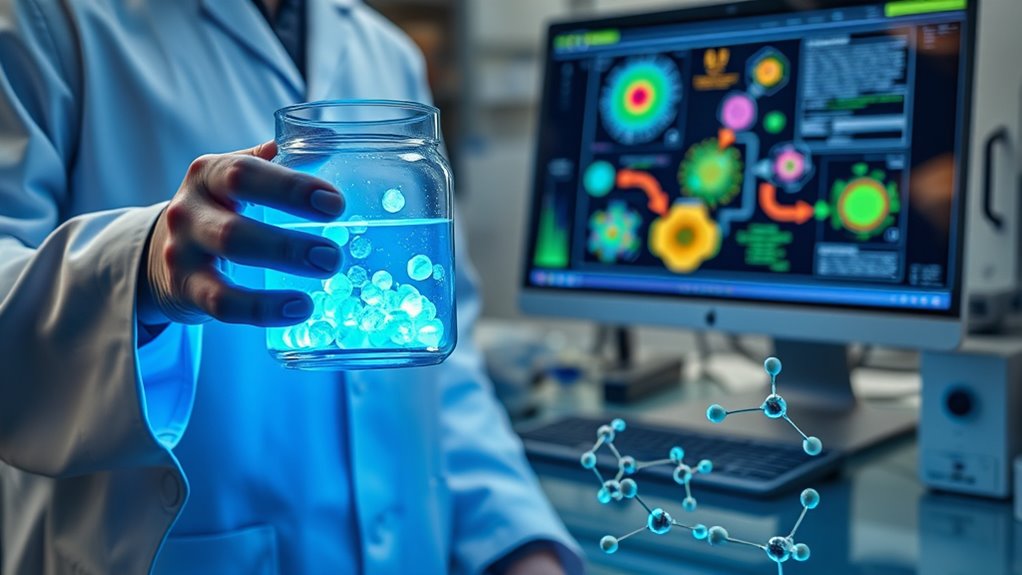
Radiation therapy not only damages tumor DNA but also activates the immune system through specific chemical pathways. It triggers DNA/RNA sensing mechanisms like cGAS-STING, leading to innate immunity activation via cytosolic nucleic acid detection.
Damaged tumor cells release DAMPs that stimulate dendritic cell maturation and antigen presentation, boosting tumor visibility. Radiation upregulates MHC class I molecules, increasing tumor antigen recognition by T cells.
Chemokines like CXCL10 and CCL5 create gradients that attract effector T cells and NK cells to irradiated sites, enhancing immune infiltration. Additionally, neoantigens exposed by DNA damage elevate tumor immunogenicity.
However, radiation also induces immunosuppressive elements, like Treg infiltration and MDSC expansion, complicating immune responses. Understanding this balance is key to optimizing radiation-induced immune activation.
Overcoming Resistance: Chemical Strategies to Improve Radiotherapy Outcomes
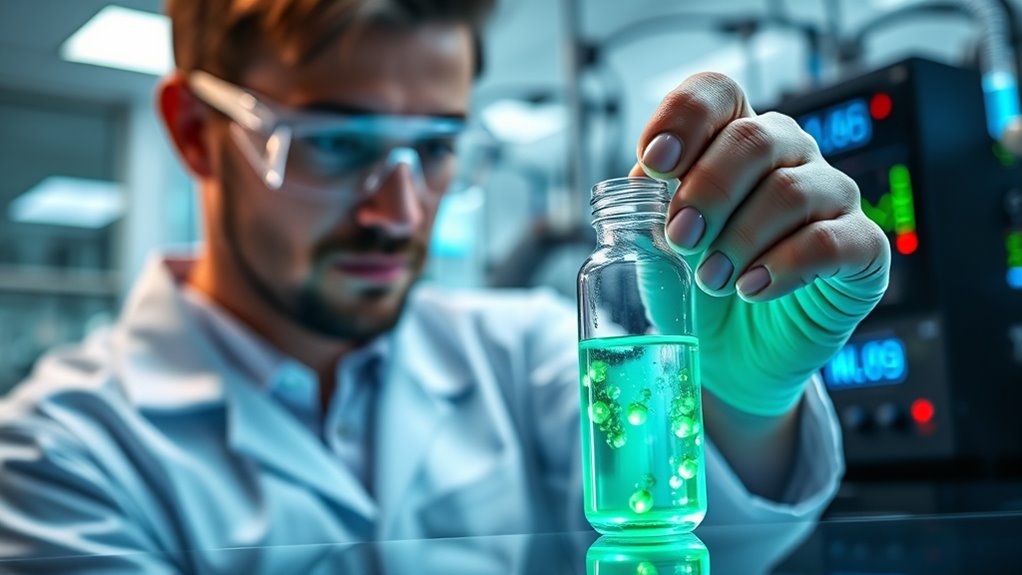
Overcoming resistance to radiotherapy requires strategic use of chemical agents that target the underlying mechanisms cancer cells use to evade treatment. You can use inhibitors to block signaling pathways cancer cells exploit, preventing their survival adaptations. RT can activate immune pathways such as the cGAS-STING pathway, which can be modulated to enhance immune responses. Combining radiation with chemotherapy or targeted therapies creates a synergistic effect, reducing resistance. Targeting immunosuppressive factors like TGF-β can restore immune responses, making tumors more vulnerable. Modulating the tumor microenvironment—such as altering the extracellular matrix or enhancing antigen presentation—can also improve outcomes. Home improvement approaches to managing the tumor environment, including optimizing oxygenation and reducing hypoxia, are increasingly recognized as complementary strategies to enhance treatment efficacy. Precision medicine approaches, tailored to your tumor’s genetic profile, allow for more effective treatments. These strategies help overcome cellular adaptations and microenvironmental barriers, ultimately increasing the efficacy of radiotherapy and improving patient prognosis.
Innovative Chemical Approaches in Next-Generation Radiation Therapies
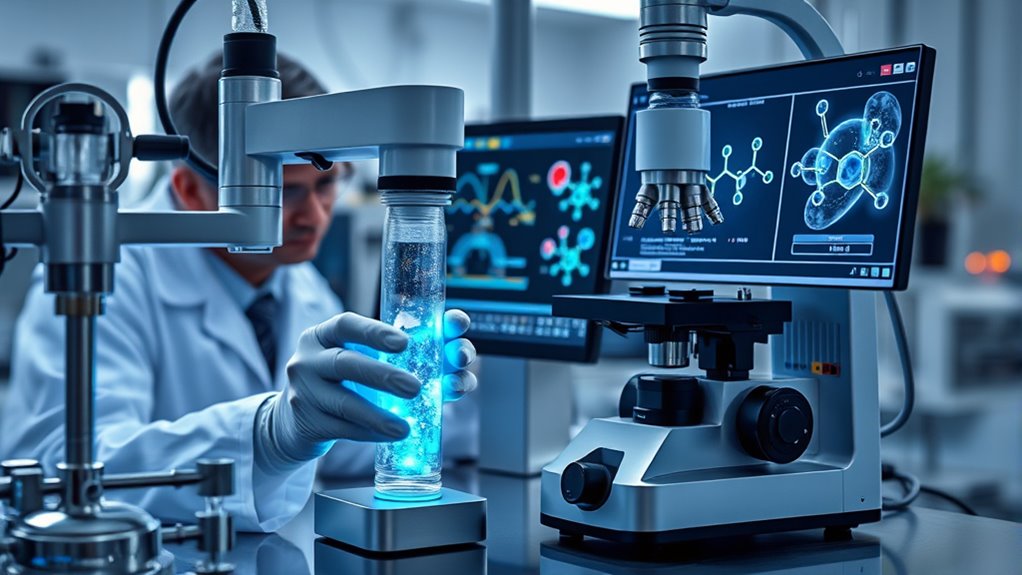
Innovative chemical approaches are transforming next-generation radiation therapies by enhancing targeting precision and treatment efficacy. Nanotechnology plays a key role, with nanoparticles engineered to boost tumor cell kill and enable image-guided delivery.
Gold nanoparticles, for example, disrupt tumor vasculature when targeted by megavoltage irradiation, causing localized damage. Thermo-sensitive liposomes allow triggered nanoparticle release deep within tumors, increasing radiosensitization.
Radioconjugates, which attach radioactive isotopes to antibodies or peptides, deliver radiation directly to cancer cells, sparing healthy tissue. Antibody-drug conjugates further refine this approach by internalizing cytotoxic payloads.
Emerging startups and space-based research accelerate these innovations, developing new materials and radiopharmaceuticals. Together, these chemical strategies aim to improve treatment specificity, reduce side effects, and pave the way for personalized cancer therapies.
Frequently Asked Questions
How Do Chemical Modifications Influence Radiopharmaceutical Stability and Targeting Specificity?
You see, chemical modifications directly impact a radiopharmaceutical’s stability and targeting. By adding stabilizing groups near the radioactive core, you reduce degradation and prevent premature radionuclide release.
These modifications also improve receptor binding and molecular recognition, enhancing tumor targeting. Additionally, backbone or amino acid changes boost metabolic stability, prolonging circulation and increasing tumor accumulation.
What Role Do Chelators Play in Radionuclide Delivery and Safety?
Think of chelators as the steadfast anchors in radionuclide delivery, ensuring your treatment stays on course. They form stable complexes with radionuclides, preventing harmful free radionuclides from escaping and causing damage.
How Can Chemical Inhibitors Enhance DNA Repair Pathways to Improve Radiation Sensitivity?
You can use chemical inhibitors to block key DNA repair enzymes like DNA-PK and ATM, preventing repair of radiation-induced damage. This makes cancer cells more vulnerable by increasing chromosomal instability and unrepaired DNA, leading to higher cell death rates.
This combination enhances tumor cell sensitivity, especially at lower doses, and can overcome resistance. This targeted approach improves treatment efficacy while sparing normal tissues.
What Are the Chemical Challenges in Developing Next-Generation Alpha-Emitting Radiopharmaceuticals?
You face chemical challenges when developing next-generation alpha-emitting radiopharmaceuticals. Limited radionuclide availability and complex production methods restrict supply.
Handling intense radioactivity requires stable formulations, while designing chelators that securely bind alpha emitters and target cancer cells is tough.
Additionally, matching chemical properties for imaging and therapy complicates development.
Variability in radionuclide half-lives demands tailored strategies, making it essential to innovate in chelation chemistry, delivery systems, and supply infrastructure.
How Does Radiochemistry Optimize Dosimetry for Personalized Cancer Treatment?
You leverage radiochemistry to optimize dosimetry by accurately measuring how radiopharmaceuticals distribute within your patient’s body. It helps you determine the right amount to administer, balancing tumor destruction with normal tissue safety.
Using advanced imaging and dose calculation tools, radiochemistry provides personalized data, allowing you to adjust treatments precisely. This approach improves outcomes, minimizes side effects, and guarantees therapies are tailored specifically to each patient’s unique biodistribution and response.
Conclusion
You now see how chemistry acts as the backbone of radiation therapy and radiopharmaceuticals. By understanding DNA damage, cell repair, and immune responses, you can appreciate how scientists are turning over a new leaf with innovative chemical strategies. These advances aim to outsmart resistance and boost treatment success. As the field evolves, it’s clear that chemistry’s role in cancer care is shaping a brighter future—proving that sometimes, the best way forward is paved with science.