Carbon nanotubes are remarkable nanomaterials made of rolled graphene sheets that offer extraordinary strength, flexibility, and electrical conductivity. They can be tailored into single-walled or multi-walled structures, with properties ideal for electronics, composites, and biomedical uses. Their high thermal conductivity also helps in heat management. However, challenges remain in controlling their synthesis and large-scale production. Exploring further reveals how these versatile materials are shaping future industries and technologies.
Key Takeaways
- Carbon nanotubes are cylindrical carbon structures with exceptional strength, electrical conductivity, and thermal properties.
- They exist as single-walled (SWCNTs) and multi-walled (MWCNTs), with properties influenced by their structure and chirality.
- CNTs are used in electronics, sensors, drug delivery, tissue engineering, and environmental remediation due to their unique functionalities.
- Their high tensile strength, flexibility, and conductivity make them ideal for reinforcing materials and creating nanoscale devices.
- Challenges include scalable, cost-effective synthesis and integration into existing technologies, impacting widespread application.
Structural Composition and Variants
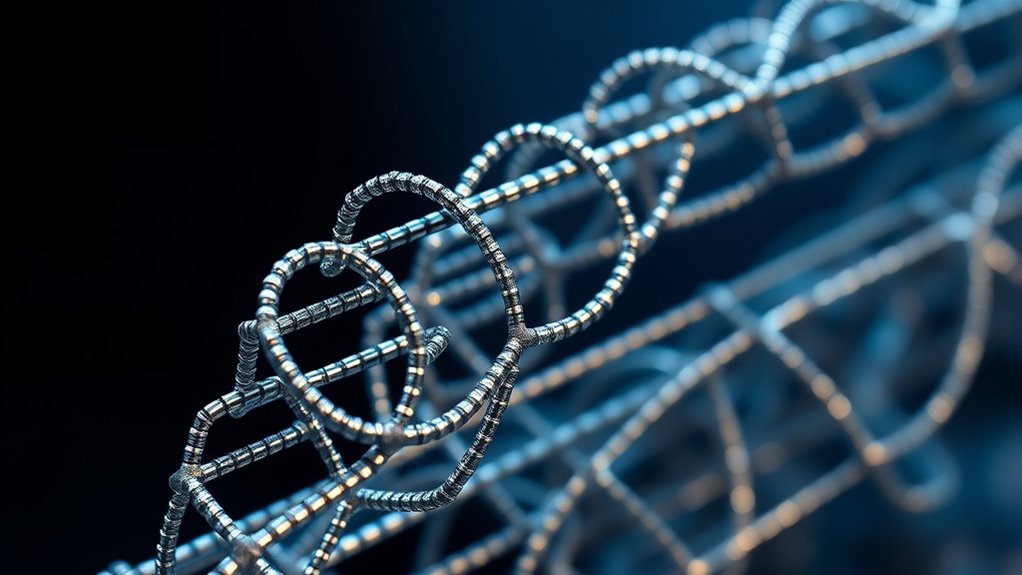
Carbon nanotubes (CNTs) are constructed from carbon atoms arranged in a hexagonal lattice, similar to graphene, which is then rolled into a cylindrical shape. This structure can be single-walled (SWCNTs) with one atom-thick graphene sheet or multi-walled (MWCNTs) featuring concentric graphene cylinders.
SWCNTs typically have diameters between 0.7 and 2 nanometers and lengths from micrometers to millimeters. The way the graphene sheet is rolled, defined by its chirality, determines whether the nanotube behaves as a metal or semiconductor.
MWCNTs, with diameters up to 100 nanometers, consist of multiple layers held together by van der Waals forces, offering enhanced mechanical strength. Variations in length, diameter, and number of walls allow CNTs to serve diverse applications across electronics, materials, and nanotechnology.
Mechanical Strength and Flexibility
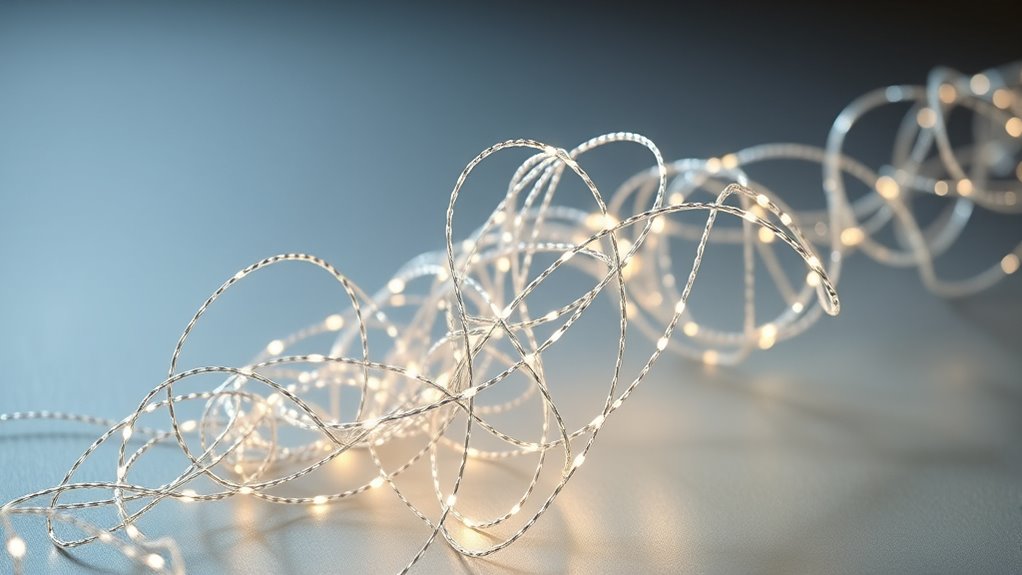
Ever wonder what makes nanotubes stand out in the domain of materials? It’s their exceptional tensile strength—up to 100 GPa, with some measurements reaching 63 GPa for multi-walled CNTs. You’ll find individual shells are 100 times stronger than steel, making CNTs the strongest known materials in tension. Their strength stems from sp² bonds supported by quantum models. When stretched excessively, CNTs undergo plastic deformation at around 5% strain, releasing strain energy and increasing maximum stretch. They’re highly flexible, forming bundles and networks that enhance mechanical resilience. Their high elastic modulus (~1 TPa) allows for significant strain tolerance, making them reliable in flexible applications—though they’re more susceptible to buckling under compression. Additionally, the self-reinforcing structure of CNTs contributes to their remarkable durability in various environments.
Electrical Conductivity and Electronic Behavior
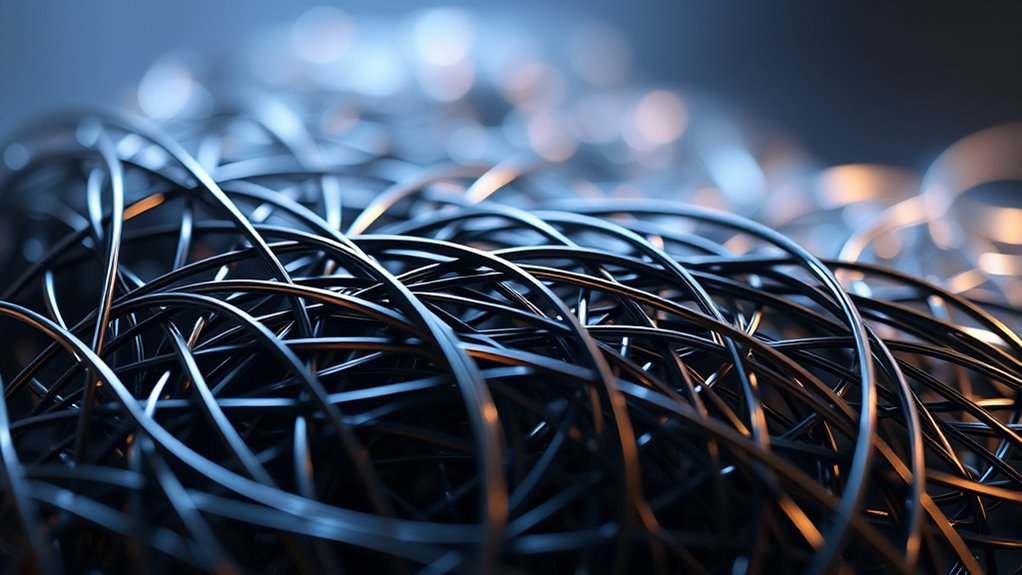
The exceptional electrical conductivity of carbon nanotubes sets them apart from traditional conductive materials, often surpassing copper’s performance. With values reaching up to 100 MS/m, CNTs can handle higher current densities, making them ideal for advanced interconnects limited by electromigration. Their high current-carrying capacity allows for the development of miniaturized yet highly efficient electronic components. Electrons travel along the nanotube’s axis, creating one-dimensional conductors with high conductance. However, their conductivity varies based on structure—some are semiconductors, others highly conductive. Junction resistance between nanotubes can reduce overall performance, especially in macroscopic wires. Factors like tunneling and contact resistance, doping, impurities, and alignment influence conductivity. Their electronic behavior, driven by quantum channels and the π-electron system, allows for efficient charge transport. Additionally, ongoing research into structure-property relationships aims to optimize their electronic applications. These properties enable CNTs to enhance conductivity in composites, interconnects, sensors, and energy storage devices.
Thermal Conductivity and Heat Management
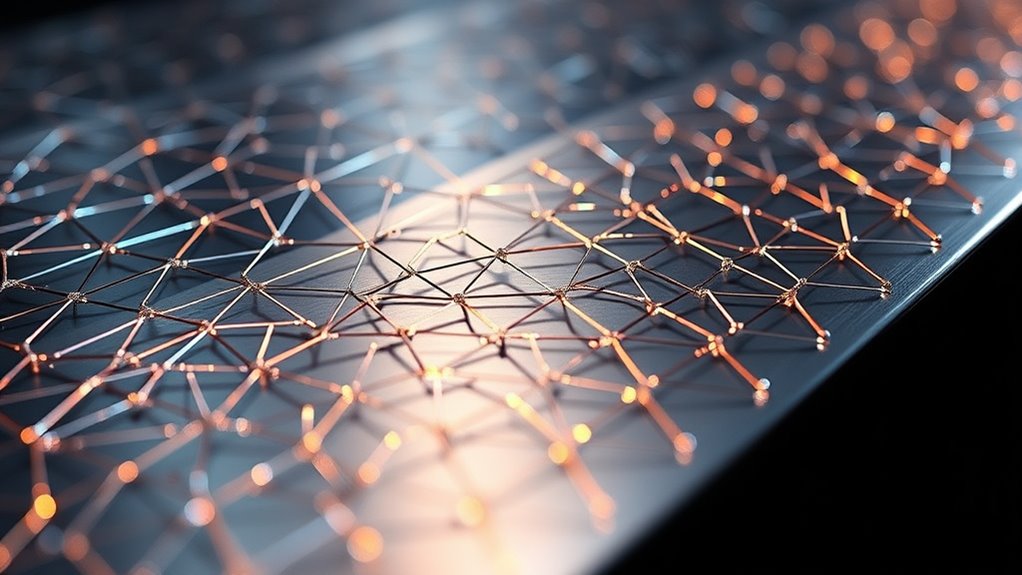
How do carbon nanotubes achieve such remarkable thermal conductivity? Their high thermal conductivities, ranging from about 2000 W/mK for single-walled to 3000 W/mK for multi-walled CNTs, stem from their unique structure. You’ll find that their strong carbon-carbon bonds enable efficient phonon transport, which carries heat along the tube. The structure influences heat conduction; double-walled CNTs can offer higher conductivity due to fewer phonon-defect scattering sites. Temperature impacts this property, as conductivity generally decreases with rising temperature because of increased phonon scattering. Additionally, the structural integrity of CNTs plays a crucial role in maintaining high thermal performance. While individual CNTs excel at heat transfer, films made from CNTs have much lower conductivity—around 15 W/mK—due to junctions and low volume filling. This makes CNTs highly suitable for heat management in electronics, composites, and thermal interface materials. Nanomaterials like CNT films can be engineered to optimize their thermal properties for specific applications, enhancing heat dissipation efficiency.
Synthesis Techniques and Structural Control
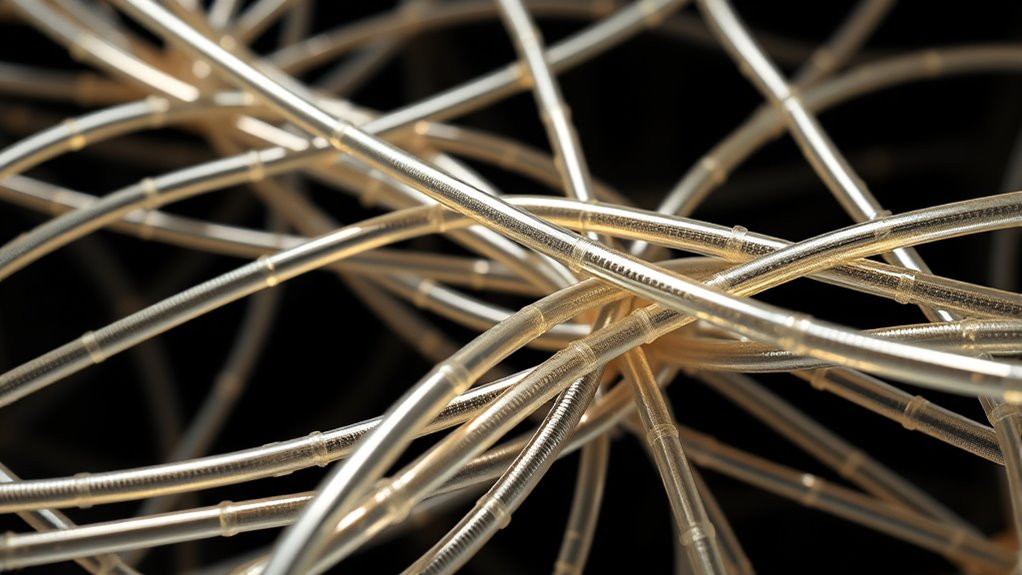
Various synthesis techniques enable the production of carbon nanotubes with specific structures and qualities, but controlling their precise features remains a complex task. The arc discharge method vaporizes graphite with an electric arc in inert gases, often producing multi-walled nanotubes. Laser ablation uses laser energy to vaporize graphite, capturing carbon fragments on collectors for high-quality nanotubes. Chemical vapor deposition (CVD) employs hydrocarbon gases and metal catalysts, allowing better control over nanotube diameter and wall number, often yielding single-walled types. High-pressure CO disproportionation and plasma methods further refine synthesis environments, influencing growth. While length and diameter can be somewhat controlled through catalyst choice and reaction conditions, features like chirality are harder to regulate. Achieving consistent purity and scalability remains challenging, but ongoing research aims to improve structural control and production efficiency. Advances in catalyst design and process optimization continue to enhance the ability to tailor nanotube features with greater precision. Additionally, growth conditions such as temperature and pressure play a crucial role in determining the final nanotube structure and quality.
Applications in Electronics and Composite Materials
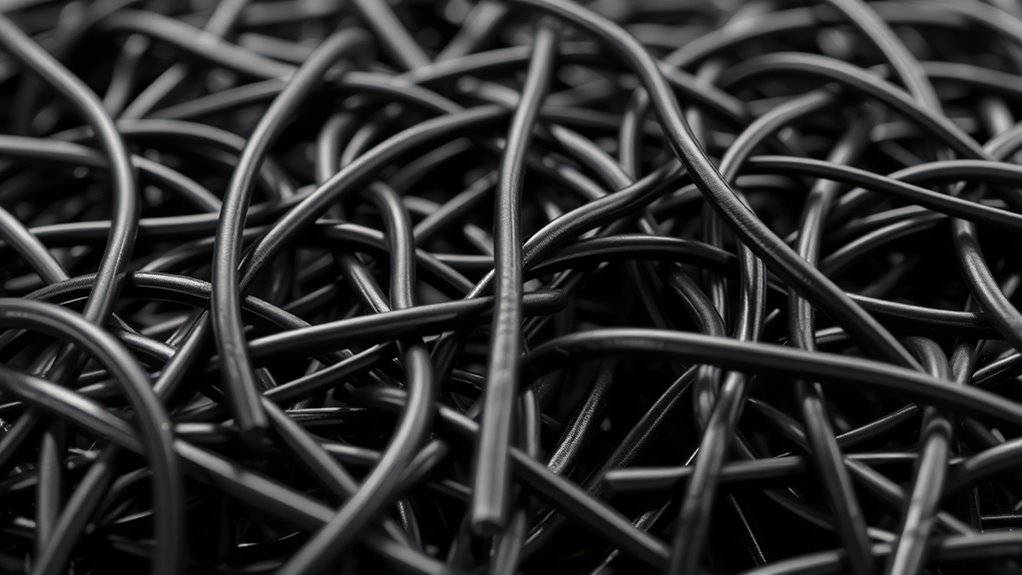
Carbon nanotubes revolutionize electronics by serving as transparent conductive films that can replace traditional materials like indium tin oxide. CNT-based TCFs deliver better conductivity, transparency, and cost efficiency, making them ideal for displays and touch screens.
They also enable flexible electronics, allowing bendable displays and wearable interfaces that maintain performance under deformation. In electronic devices and sensors, CNTs act as nanoscale switches, light emitters, and detectors, providing high sensitivity and fast response times for environmental monitoring and health diagnostics. Understanding the electrical properties of CNTs is crucial for optimizing their application in these technologies.
When integrated into polymer composites, CNTs improve mechanical strength, thermal dissipation, and electromagnetic shielding, supporting miniaturization and lightweight designs. Additionally, CNTs serve as advanced electrodes in batteries, enhancing capacity, cycle life, and rate performance, boosting overall energy storage efficiency in electronics. Furthermore, their unique electrical and mechanical properties make CNTs a promising material for next-generation electronic components and systems.
Biomedical and Environmental Uses
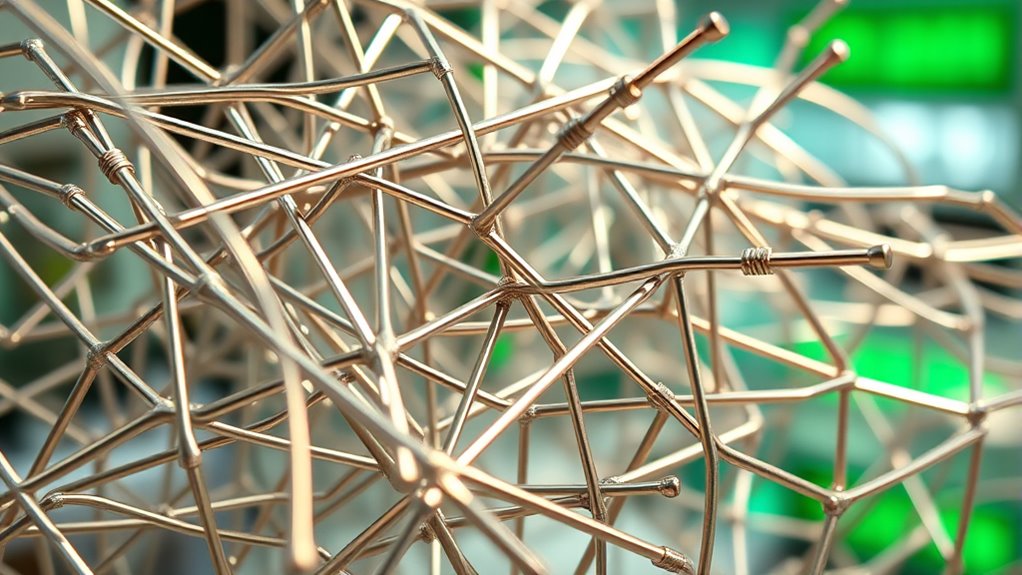
Have you ever wondered how nanotechnology is transforming medicine and environmental protection? Carbon nanotubes (CNTs) serve as powerful tools for drug delivery, gene therapy, and diagnostics. Functionalized CNTs improve solubility, biocompatibility, and membrane penetration, enabling precise targeting of tumor cells and controlled drug release. Their high surface area helps load drugs securely, protecting them from degradation. In diagnostics, CNTs enhance biosensor sensitivity and imaging resolution. They also reinforce tissue scaffolds, supporting cell growth and electrical signaling. Additionally, CNTs show antimicrobial properties, making them useful in wound dressings and surfaces. For environmental cleanup, CNTs aid in water purification by removing biological contaminants. Here’s a quick comparison:
Application | Key Benefit |
---|---|
Drug Delivery | Targeted, controlled release |
Diagnostics | Increased sensitivity and accuracy |
Tissue Engineering | Mechanical strength and electrical conductivity |
Environmental Purification | Effective biological contaminant removal |
Future Perspectives and Challenges
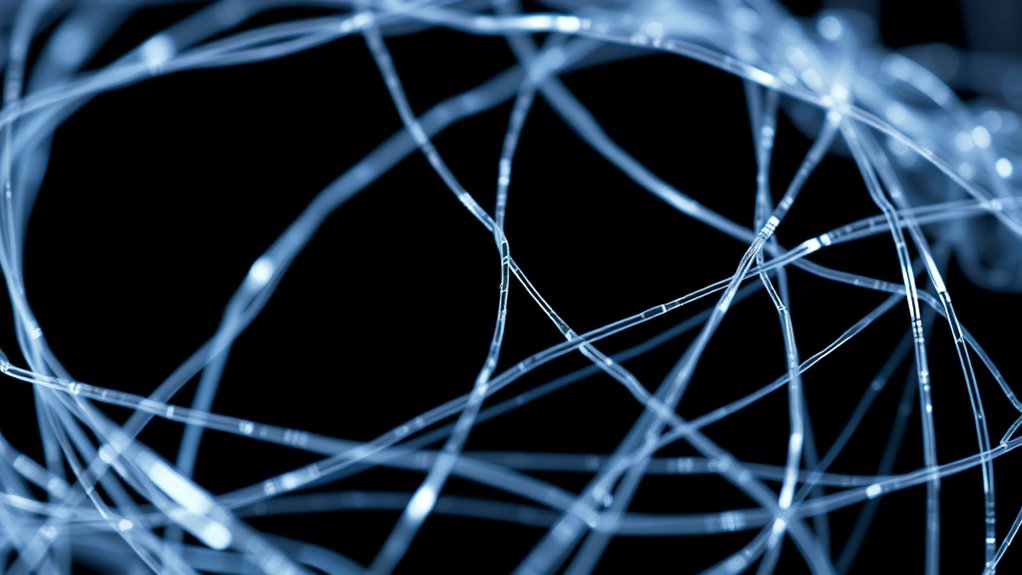
Looking ahead, the potential of carbon nanotubes (CNTs) to revolutionize industries depends on overcoming several key challenges. Achieving homogeneous synthesis remains difficult, as current methods produce heterogeneous mixtures that require complex purification.
Scaling up production while maintaining purity and uniformity is another hurdle, compounded by high costs and technical complexity. Integration issues also persist; forming reliable, conductive interfaces with metals and other materials is essential for electronic and structural applications.
Additionally, developing standardized fabrication techniques will improve quality and consistency. Economically, high production costs and infrastructure needs hinder widespread adoption.
Technologically, continued innovation in fabrication methods is vital to access CNTs’ full potential across electronics, aerospace, energy storage, and renewable energy sectors. Remote hackathons have shown how collaborative platforms can be utilized to accelerate innovation and problem solving, which could benefit CNT research and development. Addressing these challenges will shape the future of CNT development and application.
Frequently Asked Questions
How Do Defects Affect the Long-Term Stability of CNTS?
Defects in CNTs weaken their long-term stability by increasing chemical reactivity, making them prone to environmental degradation.
They also raise electrical resistance and reduce thermal conductivity, impairing device performance over time.
Additionally, defects act as stress concentrators, promoting crack growth under load, which diminishes mechanical strength.
As a result, your CNT-based materials may experience faster deterioration, decreasing their durability and reliability in long-term applications.
What Are the Environmental Impacts of CNT Production and Disposal?
Imagine a river carrying tiny, invisible pollutants—this is what CNT production and disposal can do to our environment. You face emissions of greenhouse gases and hazardous waste during manufacturing, risking contamination.
Disposal risks releasing persistent CNTs into soils and waters, harming ecosystems and bioaccumulating in food chains. Responsible handling and regulation are essential to prevent these silent threats from becoming lasting scars on our planet.
How Cost-Effective Is Large-Scale CNT Manufacturing?
You want to know how cost-effective large-scale CNT manufacturing is. By using material substitutions like low-cost polyimide, scaling up production with fluidized bed reactors, and adopting simplified methods such as open air arc discharge, you can substantially cut costs.
Automation, high-yield techniques, and process optimizations further improve economics. While challenges like quality control remain, these strategies make large-scale CNT production increasingly viable and affordable for various industrial applications.
Can CNTS Be Safely Integrated Into Human Tissues?
Think of CNTs as potential bridges between technology and biology. You can safely integrate them into human tissues by immobilizing them on substrates, which keeps the nanotubes stable and minimizes toxicity.
When CNTs are fixed in place, they promote cell survival and reduce health risks like inflammation or genotoxicity. However, avoid dispersed CNTs, as their unpredictable behavior can cause oxidative stress and long-term damage.
Proper surface modifications and controlled use are key.
What Are the Limitations of Current CNT Functionalization Methods?
You should know that current CNT functionalization methods face several limitations. They can be complex, costly, and difficult to scale up while maintaining consistency.
Achieving uniform modification is challenging, and the processes may introduce defects, reducing CNT performance. Additionally, functionalized CNTs often have decreased stability under environmental conditions and can degrade over time.
These issues hinder widespread application and require ongoing research to improve functionalization techniques.
Conclusion
You now see the stunning strength and sensational versatility of carbon nanotubes. Their remarkable resilience, radiant conductivity, and revolutionary potential promise a powerful future. As you explore their endless applications, remember that innovation ignites when imagination meets invention. Embrace these extraordinary elements, and let their limitless potential light your path forward. Together, you can harness these tiny titans to transform technology, transform lives, and transcend today’s boundaries.