Conductive polymers are special plastics that can carry electricity, combining flexibility and lightness with electrical functionality. They have conjugated molecular structures that allow electrons to move along chains, especially when doped with certain chemicals. These materials are used in sensors, solar cells, and flexible electronics. While they offer many advantages like lightweight design and tunable conductivity, challenges like stability still exist. If you keep exploring, you’ll discover how these innovative materials are shaping future technology.
Key Takeaways
- Conductive polymers are organic plastics capable of conducting electricity due to conjugated molecular structures.
- Their electrical conductivity is enhanced through doping, which introduces charge carriers like polarons and bipolarons.
- These polymers combine the lightweight, flexible properties of plastics with electrical conductivity, enabling diverse electronic applications.
- Common examples include polyaniline, polypyrrole, and polythiophene, used in sensors, energy devices, and biomedical fields.
- Despite lower stability than metals, ongoing research improves their performance, making them promising materials for flexible, sustainable electronics.
What Are Conductive Polymers?
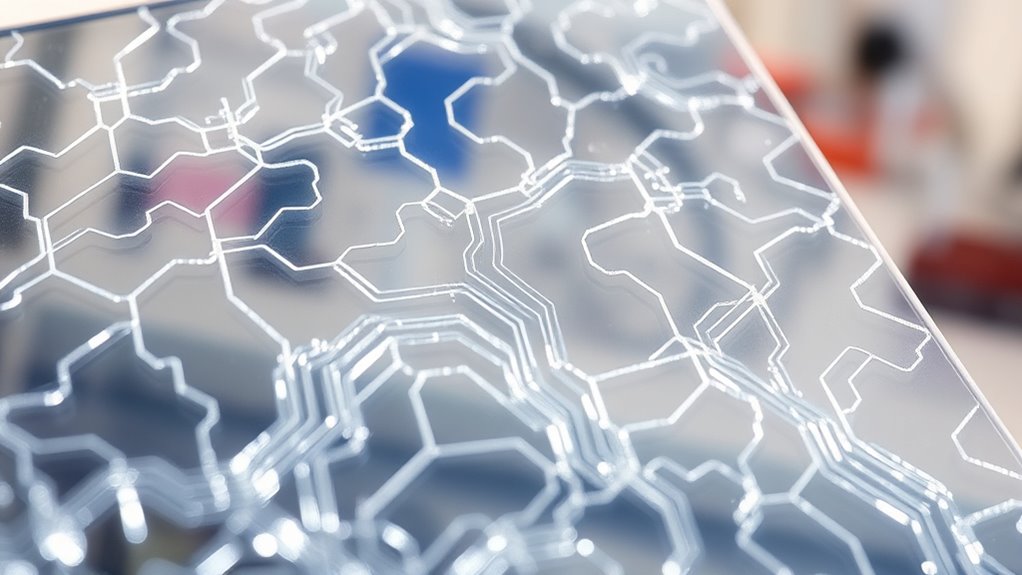
Conductive polymers, also called intrinsically conducting polymers (ICPs), are organic materials that can conduct electricity. Unlike regular polymers that act as insulators, these materials have conjugated chains with alternating single and double bonds, enabling electron movement.
Their structure includes crystalline and amorphous regions, which influence their conductivity and flexibility. Doping introduces charge carriers like polarons and bipolarons, enhancing their electrical properties.
Depending on their composition and doping level, conductive polymers can behave as metals or semiconductors. They’re typically lightweight and flexible, making them suitable for various applications.
Unlike traditional polymers, they lack thermoplasticity but can be chemically modified to improve their electrical and mechanical features. This combination of organic chemistry and electrical functionality makes conductive polymers versatile for many technological uses.
How Do They Conduct Electricity?
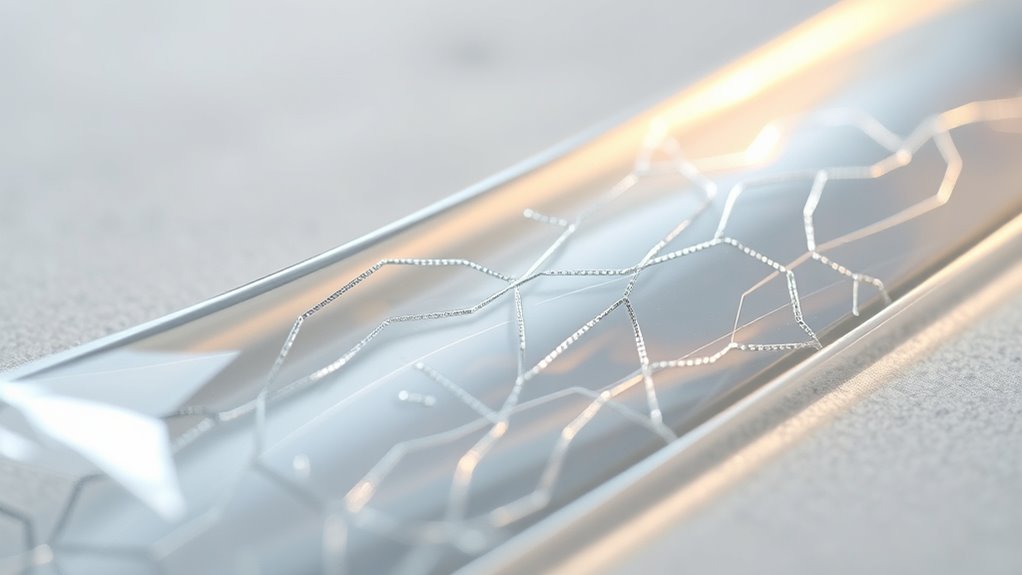
The ability of conductive polymers to carry electricity hinges on their unique molecular structure, specifically their conjugated backbone of contiguous sp² hybridized carbon atoms. This conjugation allows p orbitals to overlap, forming delocalized π electrons that can move freely along the chain. When doped through oxidation or reduction, charge carriers like polarons and bipolarons form, increasing conductivity. Doping creates new energy bands within the band gap, easing electron movement. These charge carriers hop between localized states via mechanisms like variable range hopping, involving both intra-chain and inter-chain transport. The following table summarizes how doping modifies the energy bands:
Doping Effect | Result |
---|---|
Creates new energy bands | Enhances electron mobility |
Narrows band gap | Facilitates charge excitation |
Forms polarons/bipolarons | Increases charge carriers within the polymer |
Additionally, the molecular structure of conductive polymers plays a crucial role in their electrical properties, influencing how effectively they conduct electricity.
Types of Conductive Polymers and Their Uses
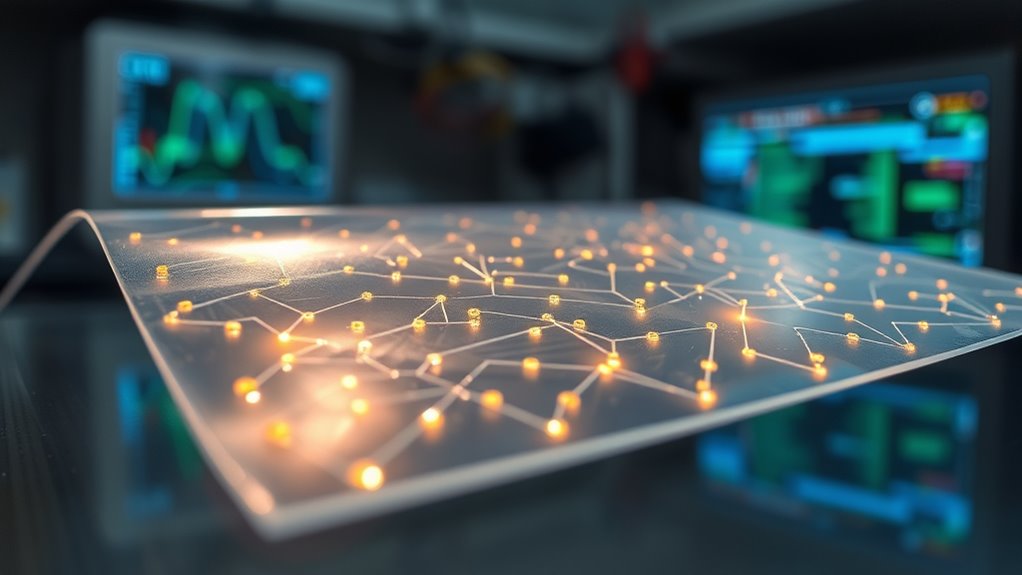
Have you ever wondered which polymers enable electronics to be flexible and lightweight? Polyaniline (PANI) is a popular choice because of its high stability, making it ideal for sensors, antistatic coatings, and corrosion protection. Polyaniline’s versatility and ease of doping allow it to be tailored for various applications, enhancing its functionality in different environments. Its widespread use reflects the growing demand for durable and adaptable conductive materials.
Polypyrrole (PPy) is valued for its conductivity and biocompatibility, so it’s used in biomedical applications like drug delivery and artificial nerves. Poly(3-alkylthiophenes) excel in solar cells and transistors thanks to their efficient charge mobility. Regional knowledge of different polymers’ properties helps in selecting appropriate materials for specific technological needs.
Polythiophene (PT) stands out for its flexibility, making it suitable for solar cells and photovoltaic devices. Although polyacetylene was the first conductive polymer, it’s less stable and less used today. These polymers are transforming electronics, energy, and biomedical fields with their unique properties.
Advantages and Challenges of Conductive Polymers
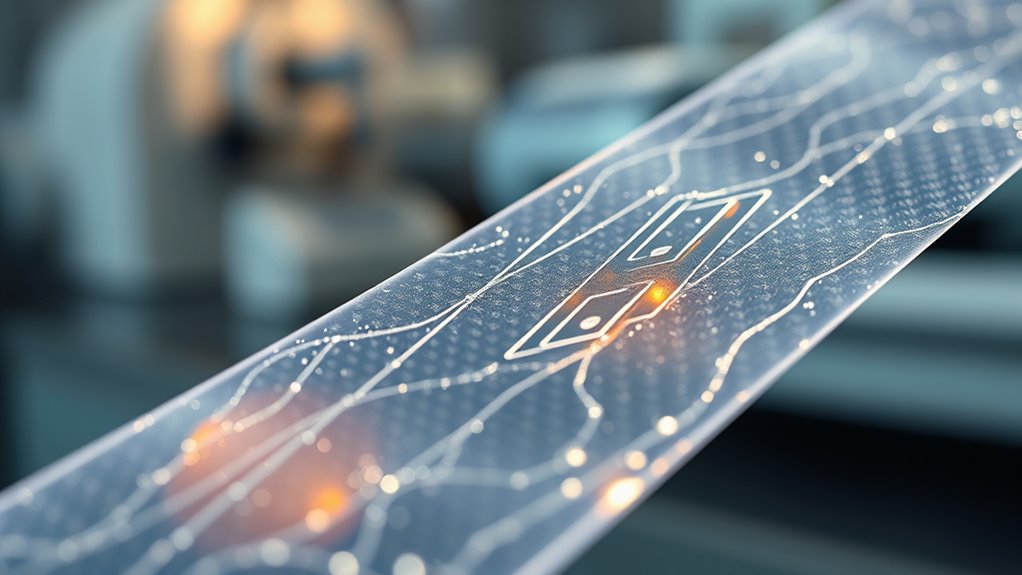
While the unique properties of conductive polymers have opened up exciting possibilities for flexible and lightweight electronics, understanding their advantages and challenges is key to their broader application.
Their lightweight and flexible nature lets you create bendable, wearable devices that are durable under mechanical stress. They’re easy to process—mainly by dispersion—reducing manufacturing costs and enabling scalable production with printing and coating techniques.
Their electrical performance, with tunable conductivity and good environmental stability, makes them suitable for many applications.
However, challenges remain: their lower thermal stability and conductivity compared to metals limit long-term reliability. Sensitivity to moisture and oxygen can degrade performance, and integrating them into existing manufacturing processes can be complex.
Balancing conductivity, flexibility, and stability continues to be a significant engineering hurdle.
Additionally, ongoing automation in business research and development are focused on overcoming these limitations to facilitate broader adoption.
Applications in Modern Technology
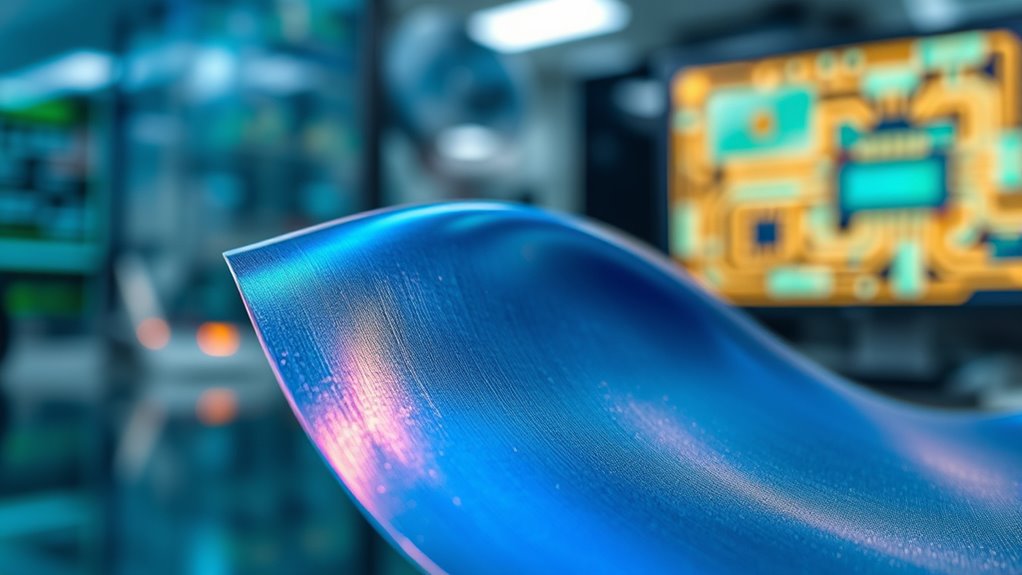
Conductive polymers play a crucial role in advancing modern technology across various fields, offering innovative solutions for energy storage, displays, and smart devices.
In energy storage, they improve supercapacitors, boost lithium-ion battery efficiency, and support energy harvesting devices like organic photovoltaics. They also enhance fuel cell performance by increasing durability. Their conductive nature enables efficient charge transfer within these devices, leading to improved performance and longevity. Additionally, the development of aesthetic wall organization systems can incorporate conductive polymers to create visually appealing yet functional design elements.
In electronic displays, conductive polymers serve as transparent electrodes in OLEDs and organic solar cells, enabling flexible, lightweight, and transparent screens. They replace traditional materials in electrochromic devices, allowing color changes with electrical stimuli.
For smart materials, they power wearable electronics, biosensors, and artificial muscles, integrating technology into fabrics and healthcare.
In communication and security, they provide antistatic properties, electromagnetic shielding, and advanced sensors, shaping the future of modern electronic systems.
Recent Advances and Future Directions
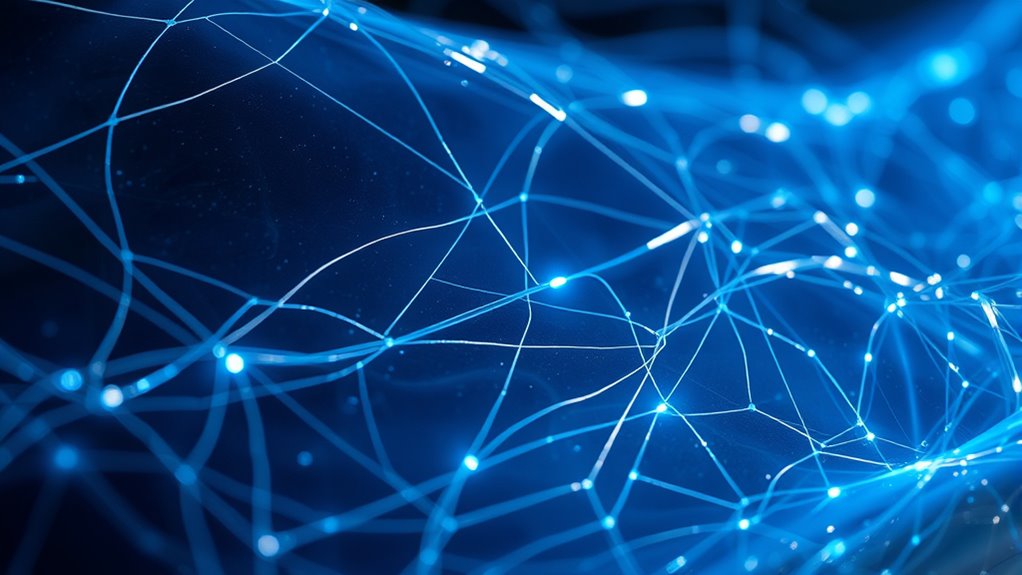
Recent breakthroughs in synthesis and fabrication techniques are transforming conductive polymers into more versatile and high-performance materials. You now have access to a wide array of methods, including chemical oxidation, electrochemical polymerization, vapor phase, and electrospinning, which optimize properties for specific applications. These methods allow precise control over the polymer morphology and doping levels, enhancing their functional capabilities. Hybrid composites combining conducting polymers with carbon nanotubes, graphene, metal oxides, and transition metals further enhance conductivity, strength, and environmental stability. Additive manufacturing allows precise, customized structures, while nanostructuring and hybridization improve electrical and optical tunability. Advances in scalable fabrication] are making these materials more accessible for commercial use. These innovations enable conductive polymers to meet the demands of advanced energy storage, sensors, photocatalysis, corrosion protection, and biomedical devices. As research continues, expect future directions to focus on scalable fabrication, improved stability, and integrating these materials into emerging technologies.
Comparing Conductive and Traditional Polymers
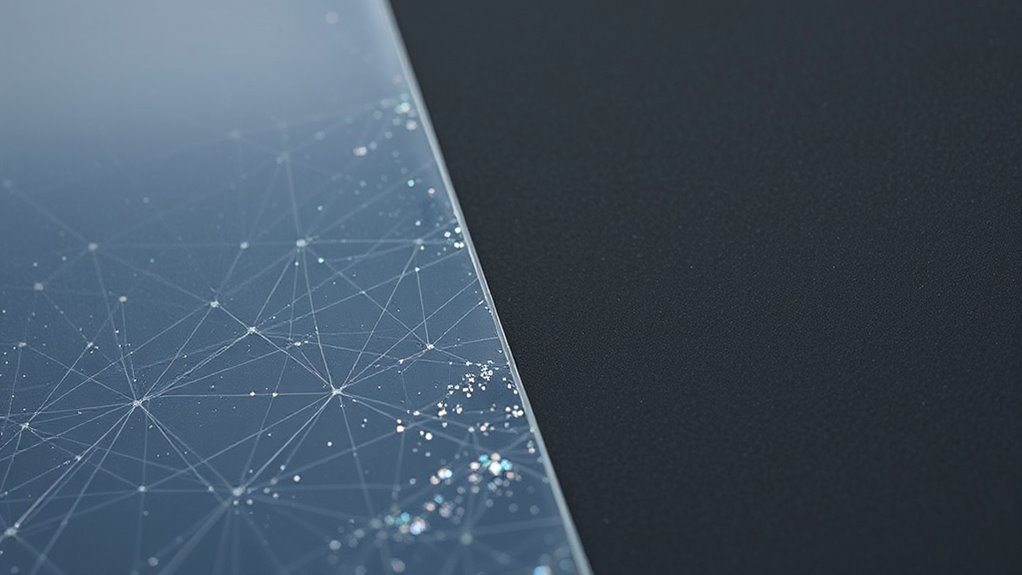
The advancements in synthesis and fabrication techniques have expanded the capabilities of conductive polymers, making it important to understand how they differ from traditional polymers.
Conductive polymers have conjugated molecular backbones that enable electron delocalization, allowing them to conduct electricity when doped. In contrast, traditional polymers consist of saturated sigma bonds with low electron mobility, making them insulators.
Conductive polymers behave like semiconductors, with tunable conductivity based on doping levels, whereas traditional polymers require metal fillers to gain conductivity.
Mechanically, both are flexible and lightweight, but conductive polymers also offer superior corrosion resistance and biocompatibility, opening biomedical applications.
While their production costs are generally lower than metals, they provide integrated electrical functionality, unlike traditional polymers, which mainly serve as insulators or structural materials.
Additionally, ongoing research into AI safety measures and the vulnerabilities of AI models highlights the importance of developing secure and reliable materials for future electronic and biomedical devices.
The Potential Impact of Conductive Polymers on Industry
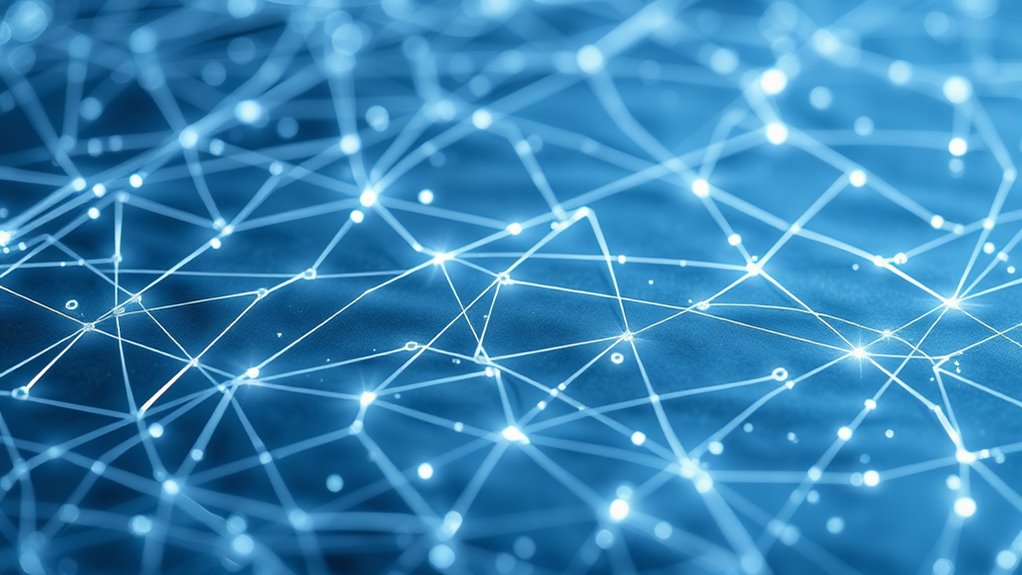
Innovative uses of conductive polymers are transforming various industries by enhancing efficiency and enabling new functionalities. You can now see their impact in organic electronics, such as OLEDs, solar cells, and transistors, boosting charge transport and device performance. High refresh rates are essential in some applications, ensuring faster response times and improved operation.
In healthcare and environmental sectors, biosensors and biochemical sensors offer high sensitivity for detecting substances, improving monitoring accuracy.
Conductive polymers also protect electronics through antistatic coatings and EMI shielding, ensuring reliability and safety.
They enable lightweight, flexible energy storage devices like supercapacitors and renewable energy solutions like solar cells, reducing costs and dependence on scarce materials.
In electronics manufacturing, they provide corrosion resistance and support flexible, printable circuits, streamlining production.
These advances position conductive polymers as pivotal in reshaping industries toward smarter, more sustainable, and efficient technologies.
Frequently Asked Questions
How Do Doping Processes Affect Conductive Polymer Stability Over Time?
Doping processes influence the long-term stability of conductive polymers by creating charge carriers that can degrade or migrate over time. If you use oxidative doping, you’ll get more stable pathways, but reductive doping might lead to instability due to environmental sensitivity.
High dopant concentration and environmental exposure can cause structural strain, dopant leakage, or degradation, reducing conductivity.
To improve stability, you should consider molecular engineering and protective strategies like crosslinking or embedding into stable matrices.
Can Conductive Polymers Be Recycled or Reused Effectively?
Imagine transforming discarded electronics into valuable materials—this is what recycling conductive polymers offers. You’re able to break them down chemically or extract them, making reuse possible.
While challenges like disassembly and variability exist, advances in solvent extraction and chemical recycling show promise.
What Environmental Factors Influence the Conductivity of These Polymers?
Environmental factors play a pivotal role in the conductivity of these polymers. You should consider how temperature changes can disrupt charge transport or cause brittleness.
Humidity may lead to water absorption, swelling, or hydrolytic degradation, which affects ionic and electronic conduction.
Additionally, dopant stability and type influence environmental resistance.
Mechanical stresses like stretching or cracking can alter chain alignment and pathway continuity, ultimately impacting the polymer’s ability to conduct electricity effectively.
Are There Biocompatible Conductive Polymers Suitable for Medical Implants?
Think of biocompatible conductive polymers as the bridge builders between technology and biology. You’ll find materials like PEDOT, which offers high conductivity and gentle interaction with tissues, making them suitable for implants.
These polymers can be tailored into various forms, from hydrogels to nanowires, to fit specific medical needs. By choosing the right combination, you can create implants that seamlessly integrate with the body, enhancing healing and functionality.
How Do Mechanical Properties Limit the Use of Conductive Polymers in Structural Applications?
Mechanical properties limit your use of conductive polymers in structural applications because they often have low tensile strength and are brittle, making them prone to cracking under stress.
Their microstructure and synthesis conditions influence strength but don’t fully solve these issues.
Environmental factors like moisture and UV exposure further degrade their durability.
Additionally, improving mechanical strength usually reduces electrical conductivity, creating a tough trade-off that restricts their effectiveness in load-bearing roles.
Conclusion
In the future, conductive polymers will revolutionize everything from smartphones to space travel, transforming how you interact with technology daily. Their unique ability to combine flexibility, conductivity, and lightweight properties will make traditional materials seem outdated overnight. Imagine a world where every device is smarter, more efficient, and almost magic—powered by these incredible plastics. Embrace this groundbreaking innovation, because conductive polymers aren’t just the future—they’re the key to accessing a universe of limitless possibilities.