Metamaterials are engineered structures that manipulate electromagnetic, acoustic, and thermal properties beyond natural limits. By designing tiny internal architectures inspired by nature or creating entirely new geometries, you can achieve effects like negative refraction, cloaking, and advanced wave control. These materials depend on precise micro and nano-scale designs, overcoming manufacturing challenges to *unveil* amazing applications. Stay with us to explore how these innovations are shaping future technologies across industries.
Key Takeaways
- Metamaterials are engineered at the micro- and nanoscale to achieve electromagnetic, acoustic, and thermal properties impossible in natural materials.
- Their unique behaviors stem from precisely designed internal structures and geometries, often inspired by biological systems.
- Advanced fabrication techniques like 3D printing enable the creation of complex architectures required for these extraordinary properties.
- By controlling interactions of waves at sub-wavelength scales, metamaterials can exhibit negative refraction, cloaking, and super-resolution effects.
- Interdisciplinary approaches combining chemistry, physics, and engineering are essential to develop and optimize these novel, artificial materials.
What Are Metamaterials and How Do They Differ From Natural Substances
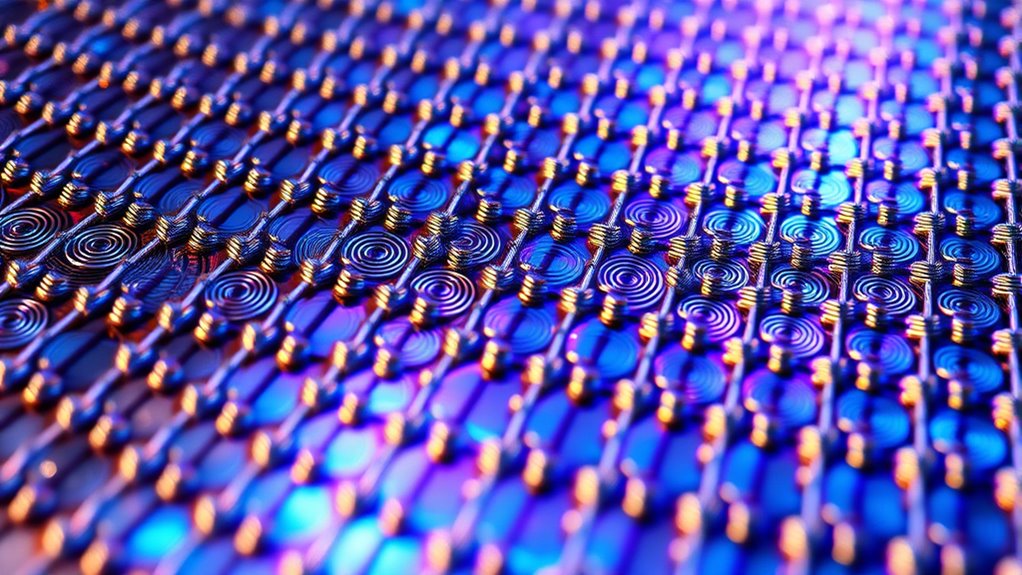
Have you ever wondered what sets metamaterials apart from natural substances? These are artificially structured materials designed to exhibit properties that don’t occur naturally. Unlike natural materials, where properties come from their chemical makeup, metamaterials rely on their unique geometry and arrangement of tiny elements—often smaller than the wavelength of waves they manipulate. Their engineered structures enable exotic electromagnetic phenomena, such as negative refraction and cloaking, which are impossible with conventional materials. This structural design allows them to control phenomena like light and sound in ways natural materials can’t. They’re part of an interdisciplinary field combining physics, engineering, and nanoscience, enabling precise tailoring of electromagnetic behavior. Moreover, the ability to engineer material properties through structural design opens new horizons for innovative technologies. Additionally, metamaterials can be considered homogeneous through homogenization, simplifying their analysis. Their versatility and ability to perform specific functions make them invaluable for innovative applications across optics, acoustics, and beyond, far surpassing traditional materials’ capabilities.
The Unique Physical Properties That Make Metamaterials Stand Out
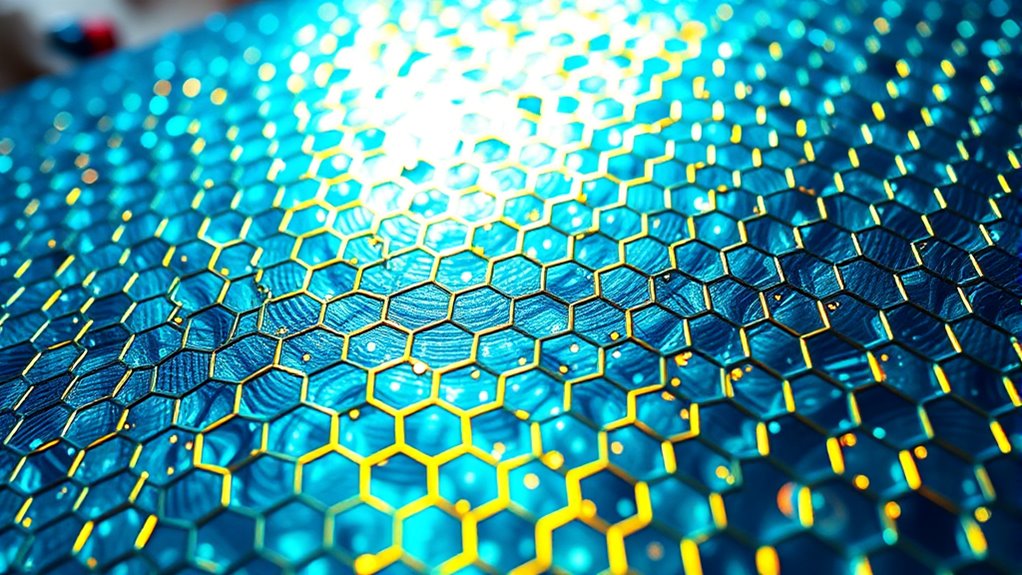
Metamaterials stand out primarily because of their extraordinary electromagnetic properties, which arise from their carefully engineered internal structures rather than their chemical composition. You can experience negative refractive index, causing light to bend in opposite directions compared to natural materials, opening new possibilities in optics. They interact effectively with electromagnetic waves smaller than their internal features, making them appear homogeneous at a large scale. This characteristic allows for advanced control over electromagnetic waves, such as focusing or diverting light with high precision. This allows for unique responses like negative electrical permittivity and magnetic permeability, enabling advanced electromagnetic control. Additionally, metamaterials can render objects invisible by guiding light around them, achieving cloaking at wavelengths shorter than visible light. Their ability to manipulate light, sound, and heat through precise microstructures makes them revolutionary, offering capabilities impossible with natural materials alone. The design of these microstructures relies heavily on principles from metamaterial engineering, which integrates concepts from physics, chemistry, and materials science to tailor properties uniquely suited to specific applications.
How Internal Structures Define Metamaterial Behavior
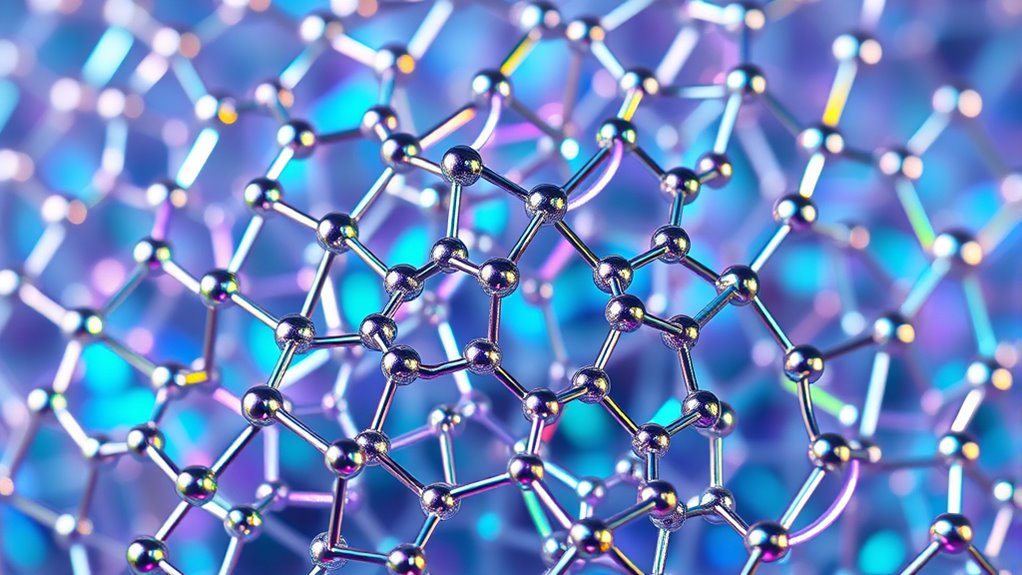
The internal structures of metamaterials are fundamental in shaping their unique behaviors, as their specific designs directly influence how they interact with waves and forces. When you craft sub-wavelength architectures, you control wave interactions—like refraction or vibration damping—by adjusting meta-atom dimensions. Overview of Mechanical Metamaterials: Properties derived from internal structural geometry. Geometrical arrangements, such as honeycomb or origami-inspired patterns, determine properties like negative stiffness or Poisson’s ratio. Periodicity amplifies emergent behaviors but can limit tunability unless active elements are added. Topological optimization balances conflicting parameters, optimizing performance. Integrating multiple materials creates hybrid structures that adapt reflection and transmission. Microstructural features like local resonance induce frequency-specific energy dissipation, while dynamic, reconfigurable designs enable real-time stiffness adjustments. These internal configurations are key to accessing the extraordinary behaviors characteristic of metamaterials, and ongoing research in adaptive materials continues to expand their potential applications.
Cutting-Edge Applications in Optics, Acoustics, and Beyond
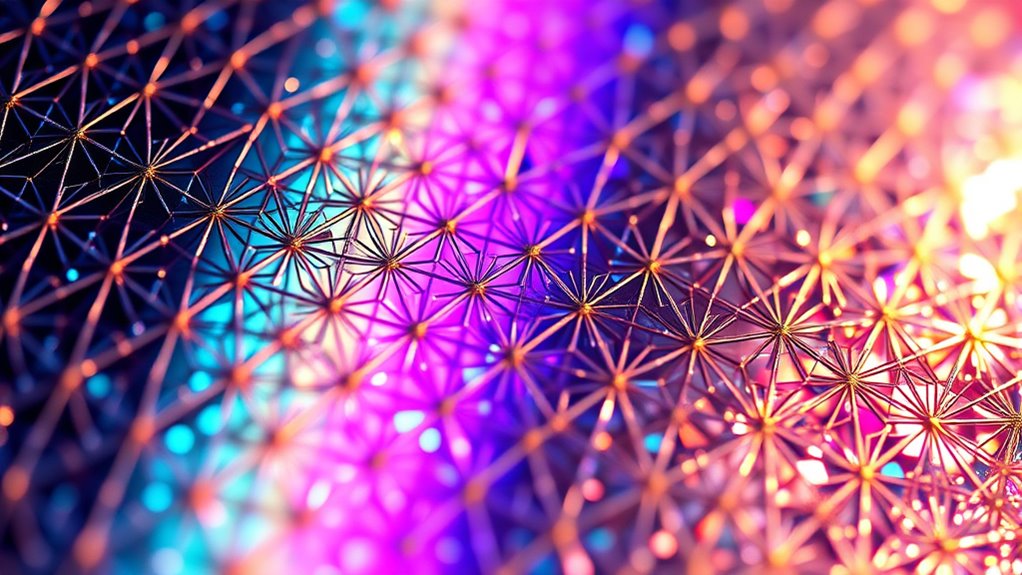
Advances in optical and acoustic metamaterials are transforming how you control light and sound, opening new possibilities across various fields. In optics, they enable negative refractive indices, creating superlenses that surpass diffraction limits, and facilitate cloaking by bending light around objects. Metamaterials can be engineered to exhibit unique electromagnetic properties that do not occur naturally, allowing for unprecedented control over light propagation. Plasmonic metamaterials boost light-matter interactions for better biosensing and photonic devices. Tunable metamaterials respond to external stimuli, making adaptive optics and switches possible. They also support extraordinary light transmission through tiny apertures for high-efficiency filters and sensors. Additionally, metamaterials’ capacity for tailored electromagnetic responses enhances their application in various innovative technologies. In acoustics, metamaterials manipulate phonons, allowing precise control over sound waves for focusing and noise reduction. They enable underwater ultrasonic focusing, acoustic diodes, and broadband sound absorption. These innovations are revolutionizing imaging, sensing, noise control, and even medical and environmental applications.
Design Strategies: Mimicking Nature and Innovating New Structures
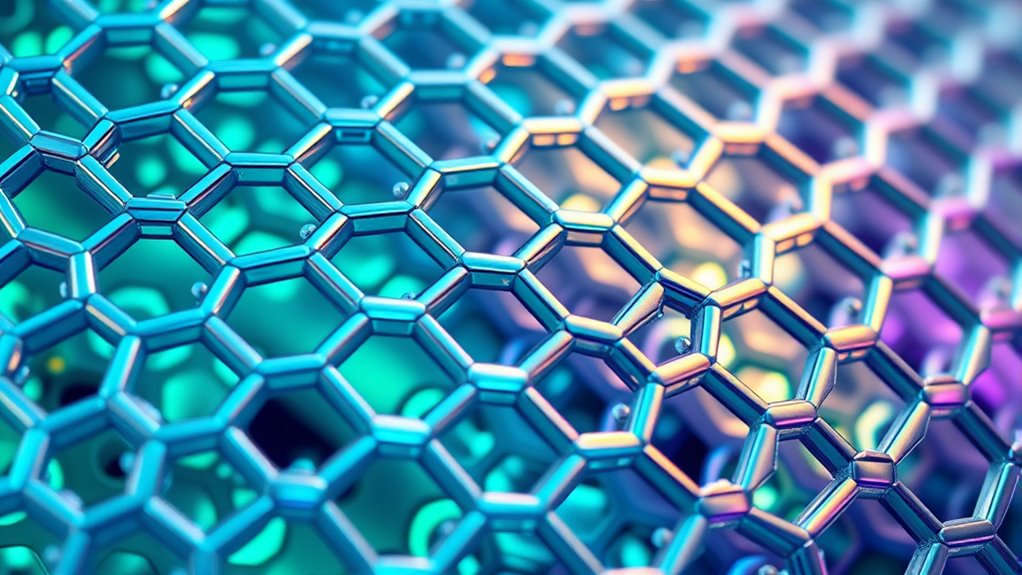
Design strategies for metamaterials often draw inspiration from nature’s remarkable structures and mechanisms. You can mimic biological systems like cell walls, exoskeletons, or spider silk to develop robust, efficient designs. Natural structures offer models for superior elasticity and resilience, as seen in abalone shells or spider silk. Developed to imitate natural processes for solving complex optimization problems. You might also incorporate adaptive mechanisms, similar to how bones repair and strengthen over time. Hierarchical structures found in biological systems can be replicated to optimize mechanical performance. To refine these designs, you can use nature-inspired algorithms such as evolutionary or genetic algorithms, which mimic natural selection. Utilizing biomimetic materials can further enhance the functionality and durability of these structures. Combining these approaches with advanced manufacturing techniques like 3D printing allows you to create complex, biomimetic architectures. This synergy opens new horizons for innovative, high-performance metamaterials rooted in nature’s time-tested solutions.
Material Choices and Their Impact on Metamaterial Functionality
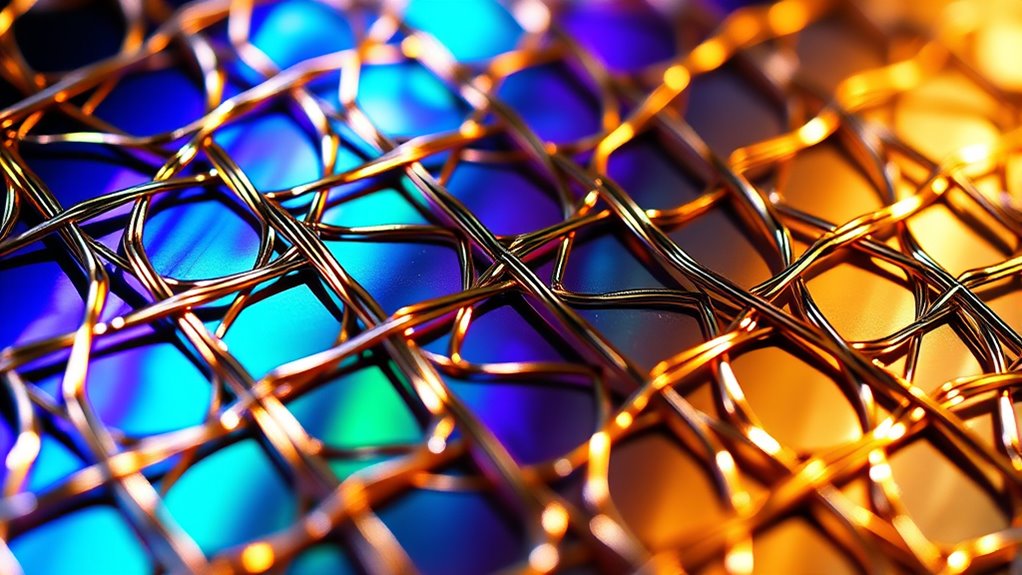
Choosing the right materials is vital because their electromagnetic properties directly influence a metamaterial’s functionality. Metals like gold and silver provide plasmonic resonance, enabling selective absorption and emission, indispensable for optical applications. Advancements in nanostructure techniques have allowed for precise engineering of these properties at the nanoscale, enhancing control over electromagnetic interactions. The use of nanostructured materials engineered at sub-wavelength scales ensures effective medium behavior. Dielectrics with contrasting permittivity allow you to manipulate effective refractive indices, shaping wave propagation. Combining metals with dielectrics creates composite structures that control electromagnetic waves anisotropically across various dimensions. The permittivity (ε) and permeability (μ) determine the metamaterial’s refractive index, enabling exotic effects like negative refraction and cloaking when tailored properly. Material properties such as thermal stability, mechanical robustness, and compatibility with fabrication methods influence the overall performance and durability of your metamaterials, guiding your choices for specific applications.
The Role of Geometrical Precision in Achieving Desired Effects
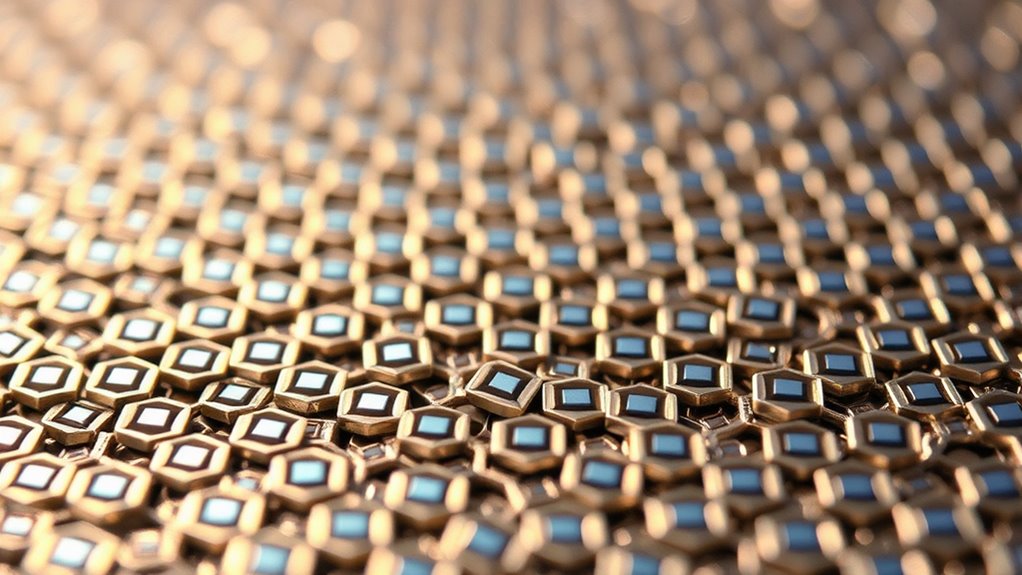
The properties of metamaterials depend heavily on the exact geometrical arrangement of their unit cells, as even slight variations can lead to significant changes in their electromagnetic, mechanical, and anisotropic behaviors. Precise geometry enables you to manipulate waves—electromagnetic, acoustic, or seismic—in ways natural materials can’t match. Additionally, vetted products and manufacturing standards are essential to ensure the accuracy and consistency required for these intricate structures. Specific designs can induce unique deformation patterns like double-sided buckling and create elastic charges that influence elastic behavior. Achieving complex 3D geometries is challenging but crucial for tailoring properties.
In optics, precise structures control light, polarization, and enable integrated photonic devices, with applications in biomedical imaging.
Mechanically, geometry influences stress distribution and nonlinear elasticity, essential for tissue engineering and drug delivery.
For anisotropic effects, exact perforations and geometrical tuning manipulate Hall coefficients and current flows, supporting theoretical predictions and experimental validation.
Overcoming Challenges in Scaling and Manufacturing Complex Structures
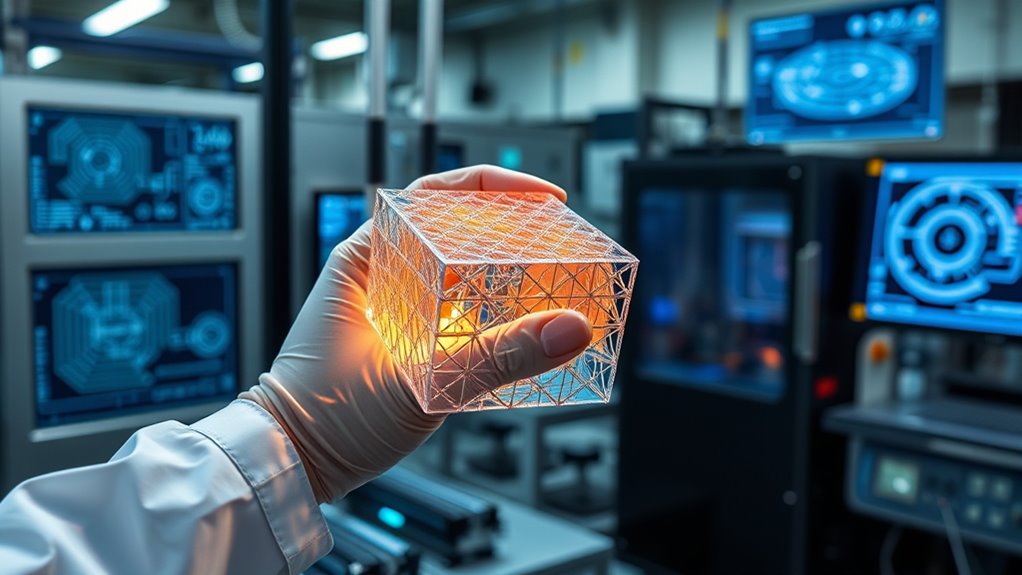
Scaling metamaterial fabrication from laboratory experiments to industrial production presents significant challenges due to the complexity of their intricate architectures. Achieving high throughput while maintaining geometric precision and cost-effectiveness is difficult. Conventional methods often struggle with complex designs, limiting large-scale manufacturing. Integrating metamaterials into existing lines requires innovations in processing and assembly across varied length scales. The table below highlights key hurdles and potential solutions:
Challenge | Solution |
---|---|
Structural complexity | Advanced additive manufacturing |
Material behavior | Developing robust, adaptable materials |
Precision control | Non-contact characterization tools |
Cost barriers | Standardized, scalable processes |
Environmental stability | Engineering for tolerance to environmental factors |
Overcoming these hurdles demands innovative approaches to guarantee reliable, scalable production of complex metamaterials. Additionally, understanding the market conditions that influence manufacturing strategies is crucial for successful large-scale deployment.
Interdisciplinary Collaboration Driving Metamaterial Advancements
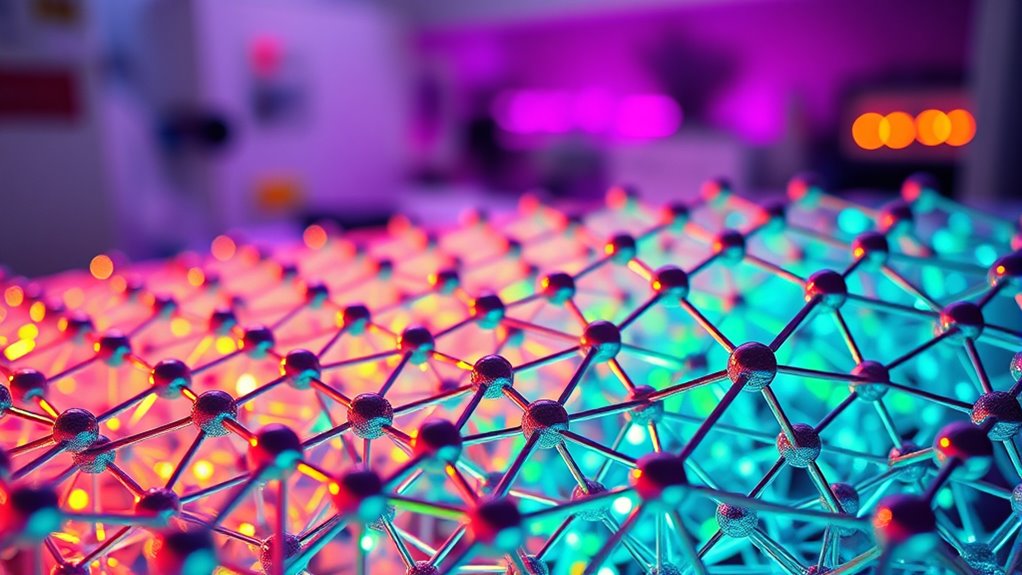
Interdisciplinary collaboration is essential for advancing metamaterials, as breakthroughs often arise at the intersection of diverse scientific fields. You’ll find that physics, chemistry, engineering, and materials science work together to develop innovative solutions. Vetted Research spans electromagnetic, quantum, mechanical, and acoustical metamaterials, benefiting from AI’s ability to optimize designs quickly. Integrating nanotechnology, biophysics, and engineering creates synergistic opportunities, pushing the boundaries of what’s possible. This collaborative approach accelerates technological applications such as quantum control, energy storage, healthcare advances, radar, and telecommunication systems.
Future Perspectives: Transforming Industries With Engineered Materials
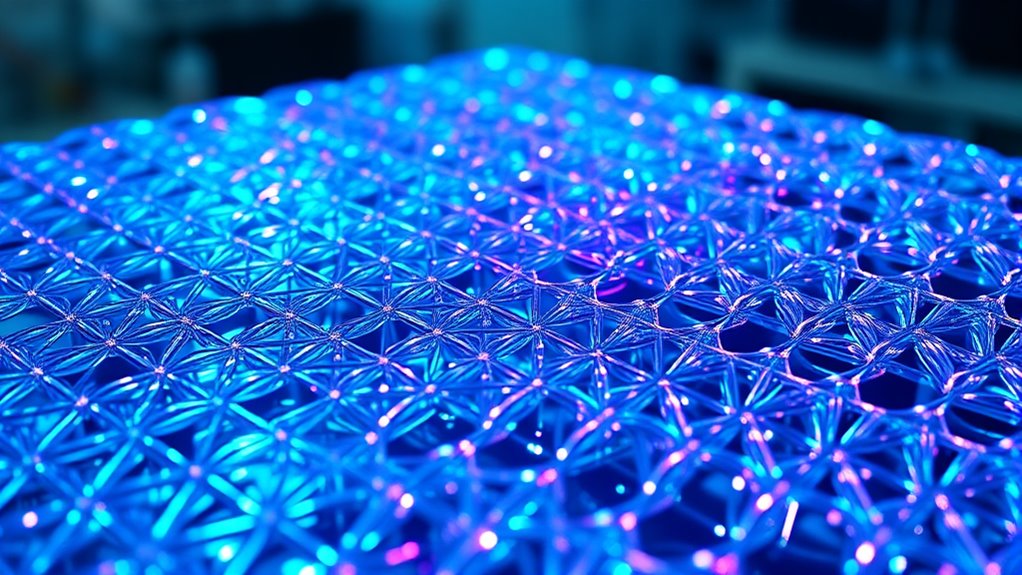
Engineered materials are poised to revolutionize industries by enabling more sustainable, efficient, and innovative manufacturing processes. Robotics and AI integration speed up material design and production, reducing defects and increasing precision.
3D printing allows for complex, lightweight, and customized geometries, expanding design possibilities. Material informatics, powered by machine learning and simulations, shorten development cycles and cut costs.
Focus on sustainability drives chemical companies to develop eco-friendly advanced materials that meet environmental standards. Automated factories and data-driven models optimize composite manufacturing for scalability and consistent quality.
Recycling innovations enable recovery of fibers and resins, supporting a circular economy and reducing waste. These advancements enhance aerospace, automotive, and civil industries by improving performance, durability, and resource efficiency, paving the way for a more sustainable future driven by engineered materials.
Frequently Asked Questions
How Do Metamaterials Achieve Properties Impossible in Natural Materials?
You see, metamaterials achieve properties impossible in natural materials by precisely designing their structures at subwavelength scales.
By arranging resonant elements like split-rings or chiral patterns, you can manipulate electromagnetic and mechanical responses uniquely.
This control enables effects like negative refraction or cloaking, which natural materials can’t produce.
Through advanced fabrication and AI-driven design, you can create these extraordinary properties, opening new possibilities in wave control and material performance.
What Are the Main Challenges in Designing Programmable Metamaterials?
When it comes to designing programmable metamaterials, you’re walking a tightrope. You face challenges like complex architectures and bulky sizes that make integration tough.
Precise control over multi-layered structures and geometric parameters adds to the difficulty.
Scaling production from nano to macro levels, ensuring material compatibility, and developing advanced control systems also pose hurdles.
All these factors require innovative solutions to turn your ideas into functional, reliable metamaterials.
How Do Metamaterials Influence Future Developments in Wireless Communication?
Metamaterials will shape the future of wireless communication by enabling more efficient, compact, and versatile systems.
You’ll see enhanced antenna performance with higher gain, wider bandwidth, and reconfigurability, making devices more reliable and adaptable. They also facilitate better signal management, boosting coverage and reducing interference.
Wireless power transfer becomes more efficient, and integrated systems support high data rates with low power consumption, paving the way for advanced 5G and beyond networks.
Can Metamaterials Be Environmentally Sustainable or Recyclable?
You might wonder if metamaterials can be environmentally sustainable or recyclable. The good news is, they can be! By utilizing bio-inspired synthesis, biopolymers, and solvent-free methods, you can create eco-friendly options.
Reconfigurable designs and material substitution reduce waste and dependency on rare resources. Plus, ongoing research focuses on developing recyclable and biodegradable metamaterials, making them a promising step toward greener, more sustainable technologies.
What Ethical Considerations Arise From Advanced Metamaterial Technologies?
Have you thought about the ethical issues linked to advanced metamaterials? You need to take into account privacy concerns, especially with cloaking and surveillance.
You also have to ensure transparency in development and prevent misuse, like military or malicious applications.
Accessibility and equitable distribution matter too, so society benefits fairly.
Don’t forget environmental impacts—your responsibility is to develop these technologies ethically, balancing innovation with social and ecological considerations.
Conclusion
So, here you are, marveling at materials nature never dreamed of creating, all thanks to clever engineering. Ironically, it’s your own hands and minds that craft these marvels, bending reality just enough to surprise even the most seasoned scientists. As you push boundaries, remember, what once seemed impossible is now just part of your toolkit. The future’s bright, and the only limit is your imagination—because nature’s rules are meant to be rewritten.