Solid-state batteries use advanced electrolytes like oxides, sulfides, and fluorides to achieve high energy density, safety, and fast charging. Manufacturing involves innovative techniques such as coating and layering to guarantee stability and performance. Industry giants and startups are pouring billions into R&D, signaling a strong market outlook—especially in electric vehicles. Challenges remain with mechanical strength and interfaces, but breakthroughs are emerging. Continue exploring to discover how these innovations could reshape energy storage and transport.
Key Takeaways
- Solid-state batteries utilize inorganic electrolytes like oxides and sulfides, offering high energy density and enhanced safety.
- Manufacturing involves layering, coating, and assembly techniques similar to traditional batteries, with automation improving scalability.
- Recent innovations include pyrochlore oxyfluoride electrolytes and composite materials, addressing interface and mechanical challenges.
- The market is rapidly growing, driven by major investments from industry leaders aiming for commercial deployment around 2026–2027.
- Key advantages include longer cycle life, faster charging, higher safety, and applications across EVs, consumer electronics, and grid storage.
Fundamentals of Solid Electrolytes and Their Composition
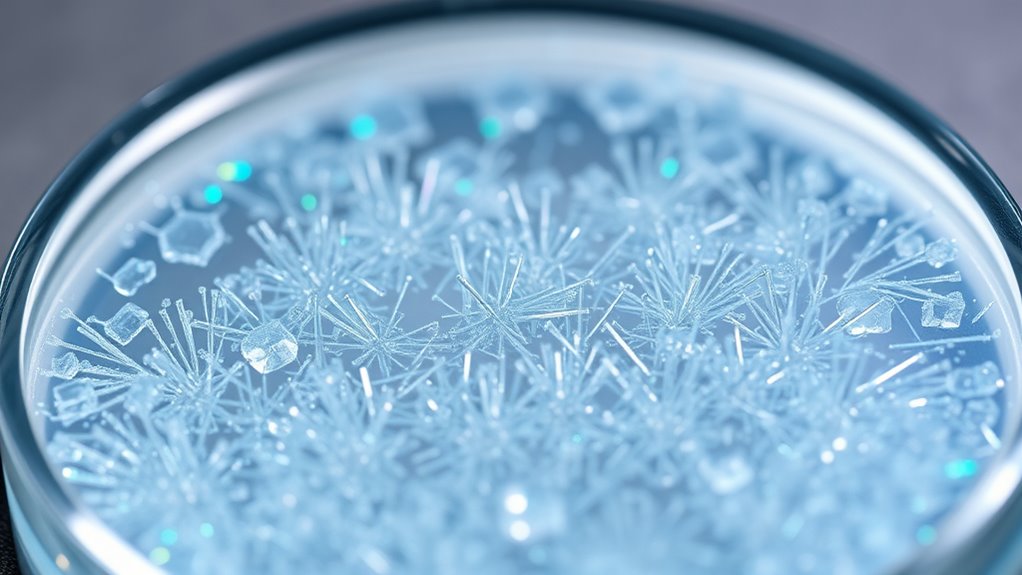
Have you ever wondered what makes solid electrolytes essential for solid-state batteries? Their key feature is high ionic conductivity, usually above 10^-4 S/cm, which allows ions to flow efficiently. You’ll find that materials like polymers, oxides, and sulfides are most common. Inorganic electrolytes, such as oxides and sulfides, offer better chemical stability and mechanical strength, helping prevent issues like dendrite formation. Oxides are known for their durability and stability, while sulfides provide higher ionic conductivity but may face stability challenges. Polymers, although less conductive, are useful in large-format batteries due to their market maturity. The composition of these electrolytes directly influences their performance, stability, and manufacturability, making them a critical component in advancing solid-state battery technology. Additionally, the Law of Attraction principles can be applied to innovate and inspire new materials and solutions within this field.
Advances in Manufacturing Techniques for Solid-State Cells
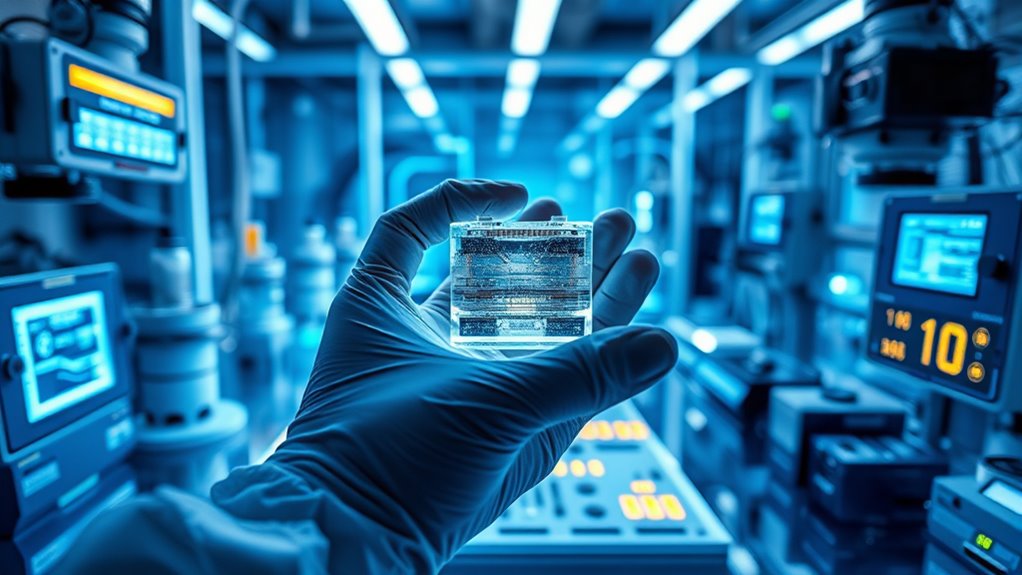
Recent advances in manufacturing techniques have markedly improved the production of solid-state cells, addressing key challenges related to material handling and layer integration. Electrode production now uses familiar processes, starting with raw materials like LiCoO₂, which are melted and coated onto current collectors, then compacted with rollers. This approach streamlines the transition from traditional lithium-ion cell manufacturing. Layer formation involves casting or extrusion, while electrode stacking replaces winding due to the stiffness of solid components, reducing deformation risks. Innovations in electrolyte production include oxide, sulfide, and polymer types, each requiring specialized coating methods and strict defect control to guarantee quality. Automation enhances consistency and speeds up assembly, with precise layer alignment and compression crucial for ideal contact. Quality control protocols are essential at every step to ensure the integrity of each cell. Finishing processes like calendering, heat treatment, and quality control ensure cell stability, sealing the manufacturing process for reliable, high-performance solid-state batteries.
Enhancing Battery Performance: Energy Density and Longevity
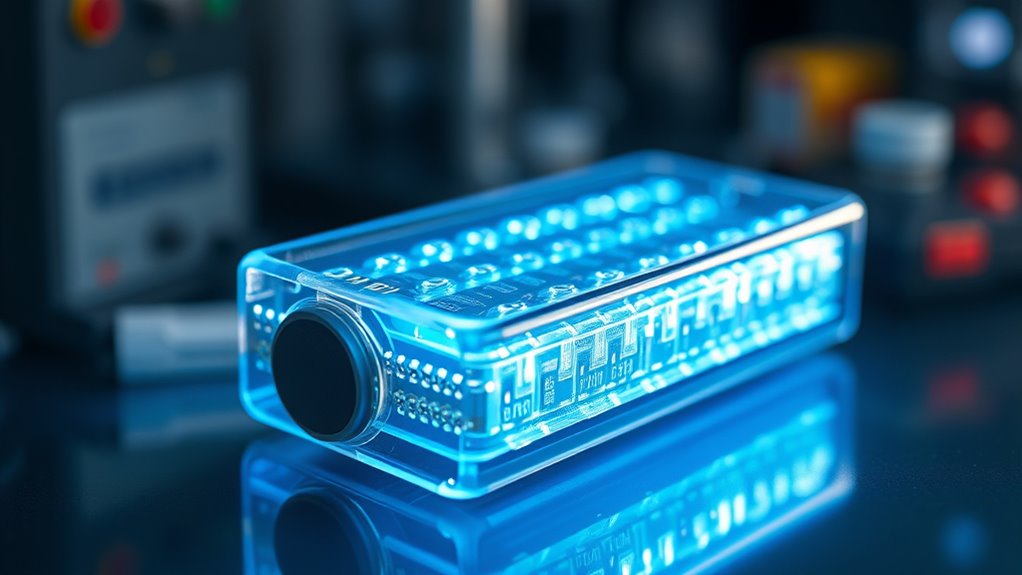
Enhancing battery performance involves increasing both energy density and longevity to meet the demands of modern applications. Solid‑state batteries offer higher energy storage, with Samsung SDI’s reaching 500 Wh/kg—nearly double that of traditional EV batteries—potentially boosting driving ranges 2 to 10 times. They’re smaller, lighter, and support design innovations that improve efficiency. Longevity is also critical; these batteries can endure 8,000–10,000 cycles, thanks to dendrite prevention and stable chemistry. They perform well at low temperatures and support rapid charging in 5–10 minutes, reducing vehicle costs. The table below highlights key performance attributes:
Attribute | Current Capability | Future Potential |
---|---|---|
Energy Density | 500 Wh/kg | Further improvements possible |
Cycle Life | 8,000–10,000 cycles | Extended durability |
Safety | Fire risk eliminated | Enhanced stability |
Charging Speed | 5–10 minutes | Faster charging |
Material Innovation | Lithium-metal anodes, solid electrolytes | New materials to optimize performance |
Additionally, advancements in Retirement Planning strategies can help investors prepare financially for the future, ensuring they can maximize benefits from their investments.
Overcoming Mechanical and Interface Challenges
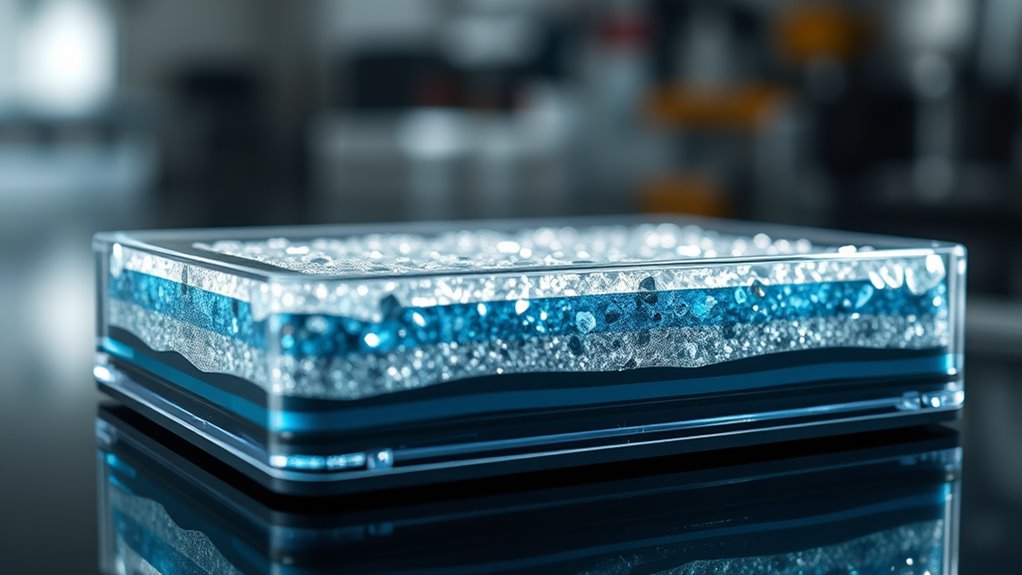
Overcoming mechanical and interface challenges is essential for advancing solid-state batteries toward commercial viability. The brittleness of ceramic electrolytes makes them vulnerable to cracking under stress and vibrations, risking internal damage. Electrode deformation during charge and discharge causes structural issues, while volume expansion can deteriorate interfaces and increase mechanical stress. Solid electrolytes are brittle, which further complicates the design of durable, long-lasting batteries. At the interface, high resistance and poor contact hinder ion flow, reducing performance and lifespan. Developing new materials like halide and sulfide-chloride electrolytes, along with advanced manufacturing techniques such as cold sintering and thin-film deposition, can improve contact and stability. In situ characterization helps monitor interface evolution, guiding improvements. Addressing these challenges reduces cracking, internal shorting, and performance decay, bringing solid-state batteries closer to reliable, large-scale application.
Applications Transforming Electric Vehicles and Beyond
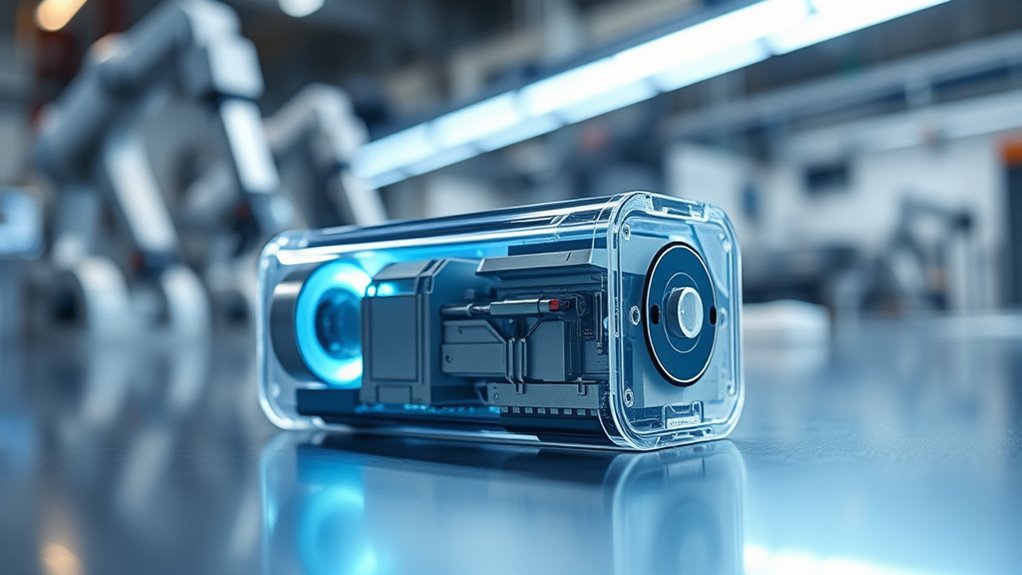
Solid-state batteries are poised to revolutionize electric vehicles by offering significant safety and performance improvements. They reduce fire risks by replacing flammable liquid electrolytes with solid ones, making vehicles safer. Their higher energy density can double range in smaller, lighter packs, while faster charging times match traditional refueling speeds. Additionally, these batteries last longer, withstanding thousands of cycles before noticeable degradation, leading to lower maintenance costs. Beyond EVs, they can enhance consumer electronics, improve grid energy storage, and support industrial power systems. As production scales, costs are expected to fall, broadening their applications. Here’s a quick overview:
Application | Benefit | Future Potential |
---|---|---|
Electric Vehicles | Increased safety and range | Market entry before 2030 |
Consumer Electronics | Improved safety and longevity | Enhanced device performance |
Grid Energy Storage | Reliability and efficiency gains | Renewable integration |
Industrial Systems | Safer, efficient power solutions | Industrial automation |
Solid-state batteries are also being developed with different variants, including semi-solid electrolytes and ceramic-coated electrolytes, which could further enhance safety and performance. These innovations could lead to more versatile applications, such as flexible or shape-conforming batteries for specialized devices. Additionally, research into advanced electrolytes is paving the way for even safer and more efficient battery designs.
Material Innovations Driving the Future of SSBs
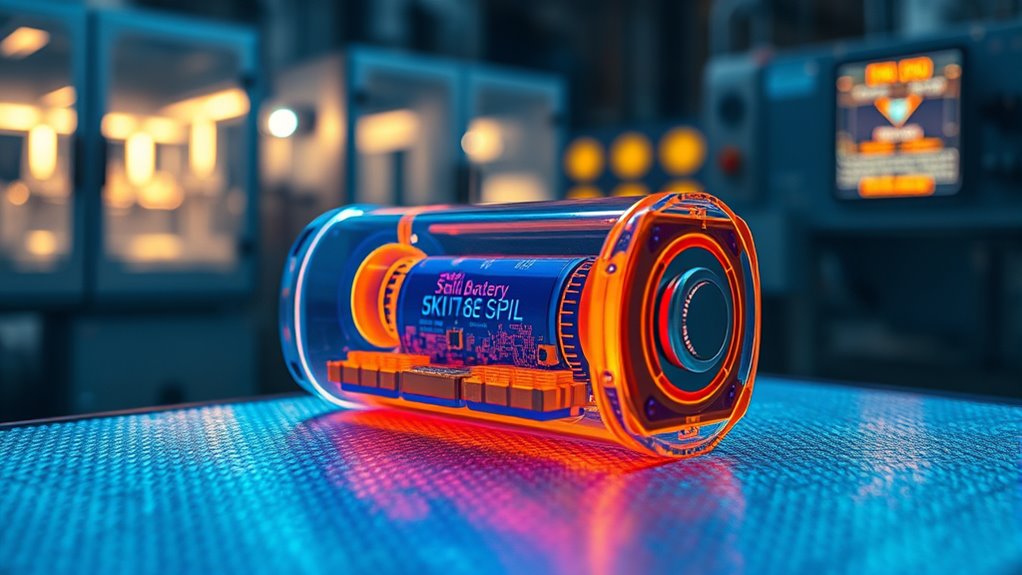
Advances in electrolyte materials are central to unfastening the full potential of solid-state batteries. Developing electrolytes with higher ionic conductivity, like sulfide, oxide, and composite types, boosts performance and safety.
Sulfide electrolytes offer conductivity comparable to liquid electrolytes but face moisture and stability issues. Oxide electrolytes excel in stability and compatibility but are more brittle and less conductive. Recent discoveries include pyrochlore-type oxyfluoride electrolytes with high ionic conductivity, opening new pathways for robust solid electrolytes. These innovations are crucial for overcoming the interfacial stability challenges that currently limit commercial applications.
Composite electrolytes combine polymers and ceramics, improving flexibility and conductivity while addressing structural challenges.
Innovations also focus on enhancing interfacial stability between electrolytes and electrodes, reducing resistance, and extending cycle life.
These material breakthroughs enable safer, more durable, and higher-performing batteries, paving the way for widespread adoption in electric vehicles and energy storage systems.
Market Dynamics and Industry Investment Trends
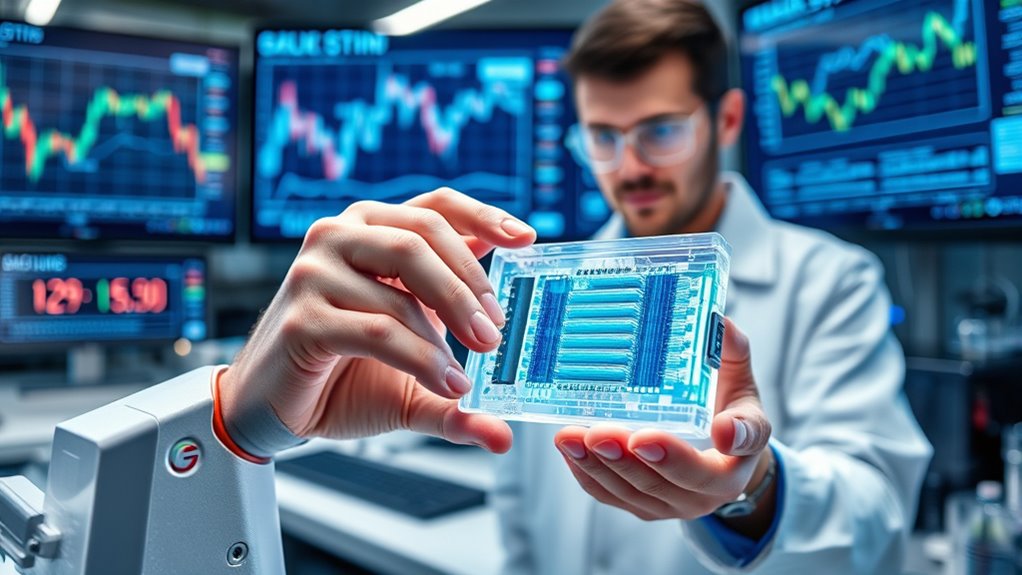
The rapid pace of innovation in electrolyte materials and battery design has sparked significant interest from industry stakeholders, fueling the expansion of the solid‑state battery market. Major investments from automotive giants like Toyota, Bosch, and startups such as QuantumScape highlight a strong focus on R&D and scaling production. Venture capital funding flows into breakthrough technologies, while partnerships accelerate commercialization. Asia Pacific leads with about 44% market share, driven by China’s manufacturing strength and EV growth. Europe and North America focus on pilot lines and energy storage, supported by favorable policies. This dynamic environment pushes the market toward a projected value of $17–24 billion by the early 2030s. The table below summarizes key industry players and regional trends:
Region | Key Focus |
---|---|
Asia Pacific | Manufacturing, EV demand |
Europe | Automotive, energy storage |
North America | R&D, pilot production |
Advancements in electrolyte materials and scalable manufacturing processes are critical drivers shaping the future landscape of solid‑state batteries.
Regulatory Environment and Policy Incentives
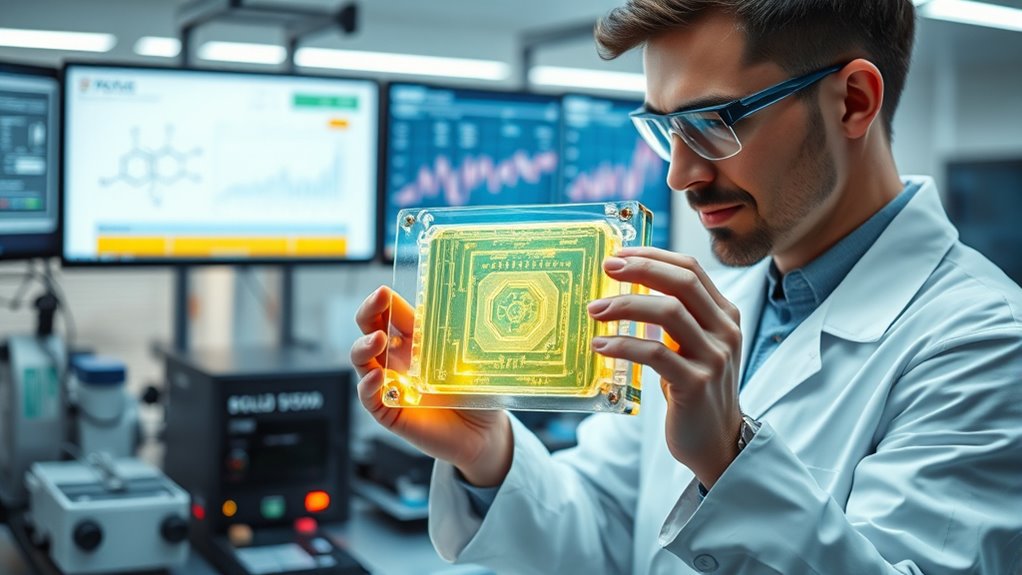
Regulatory frameworks and policy incentives play a crucial role in shaping the development and adoption of solid-state batteries. The EU’s new Battery Regulation emphasizes sustainable sourcing and recycling, supporting advancements in solid-state tech. It prioritizes responsible lithium extraction, aiming for lithium recycling rates of 70% by 2025 and 90% by 2030, while ensuring environmental and human rights protections. These policies promote demand creation, safety improvements, and environmental benefits, encouraging manufacturers to adopt solid-state solutions. Incentives for recycling infrastructure and waste management further bolster this shift. Additionally, the importance of grocery savings strategies and other consumer-focused policies can influence overall industry sustainability efforts. However, challenges like material sourcing, scalability, and cost competitiveness remain. Effective regulation and policy support are essential to build resilient supply chains, foster innovation, and accelerate market adoption of solid-state batteries, ultimately advancing cleaner and safer energy storage solutions.
Key Players and Patent Landscape in Solid-State Battery Development
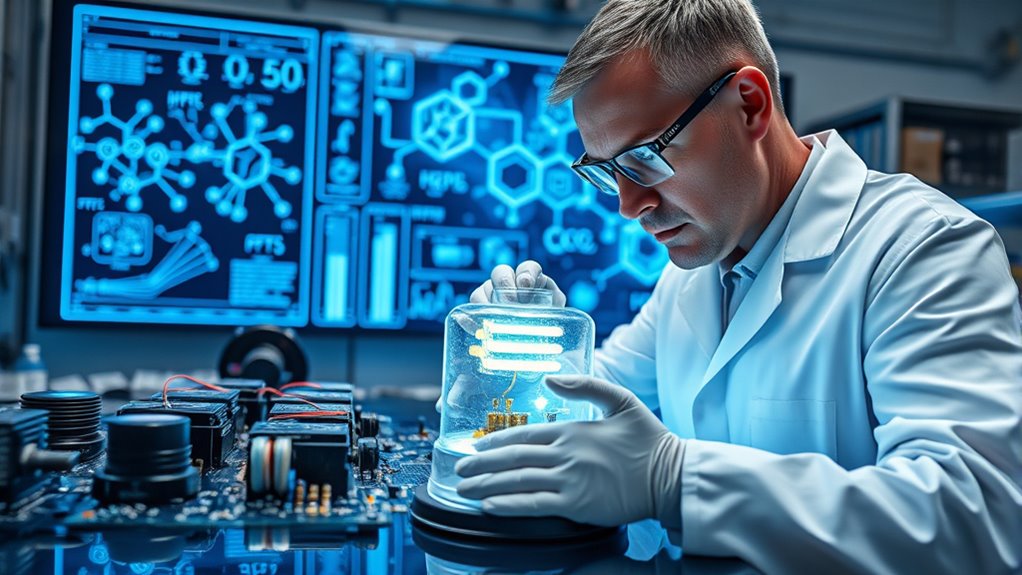
Leading companies like CATL, BYD, LG Energy Solution, Samsung SDI, and QuantumScape are at the forefront of solid-state battery development, investing heavily in research, innovation, and strategic partnerships. They’re securing patents focused on electrolyte materials, electrode designs, and manufacturing processes to protect their breakthroughs. These innovations aim to boost energy density, safety, and charging speed, pushing the industry forward. Their collaborations, such as LG with UC San Diego and QuantumScape with Volkswagen, accelerate commercialization efforts. The patent landscape is fiercely competitive, reflecting a race to dominate this transformative technology. Understanding patent strategies is crucial for assessing industry leadership and future advancements.
Future Outlook and Emerging Opportunities in Solid-State Energy Storage
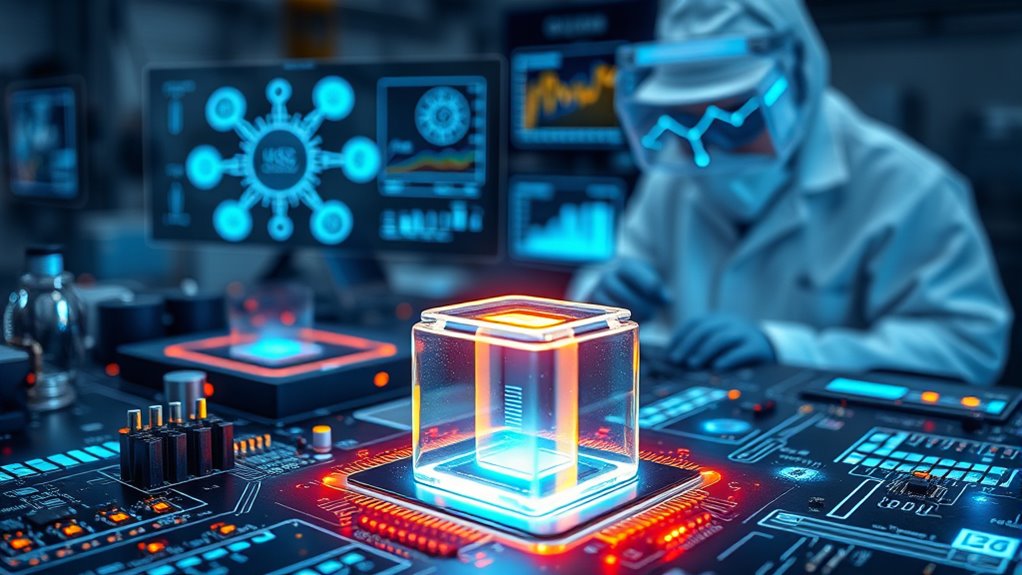
Advancements in solid-state energy storage are poised to revolutionize how you use and rely on batteries across various sectors. With higher energy density, these batteries enable longer-lasting power for electric vehicles, portable electronics, and renewable energy systems.
Their enhanced safety reduces fire and leak risks, making energy storage more reliable. Longer lifespans—up to 10,000 charge cycles—mean less frequent replacements, lowering costs. Improved temperature performance ensures dependable operation in extreme environments.
Faster charging times make energy more accessible and convenient. Emerging applications like EVs, solar systems, and microgrids will benefit from these innovations. Additionally, ongoing research into battery manufacturing techniques aims to overcome current production challenges and accelerate commercialization, expected around 2026–2027. As the technology matures, it promises to reshape energy storage, driving efficiency, safety, and sustainability across industries.
Frequently Asked Questions
What Are the Main Safety Advantages of Solid-State Batteries Over Traditional Lithium-Ion Cells?
You want to know the safety advantages of solid-state batteries over lithium-ion cells. They eliminate flammable liquid electrolytes, reducing fire risks.
They operate safely at higher temperatures, minimizing overheating.
Their solid structure resists impact and prevents dendrite growth, lowering short-circuit chances.
Plus, they last longer, degrade slower, and need less maintenance, all contributing to safer, more reliable energy storage.
How Do Solid Electrolytes Impact the Manufacturing Cost of Batteries?
You might wonder how solid electrolytes affect battery manufacturing costs. They can lower expenses through higher conductivity, reducing the need for complex cooling systems and safety features.
Using materials like sulfides or garnets may raise material costs but can streamline production with emerging methods like aerosol deposition.
What Are the Current Limitations in Scaling up Solid-State Battery Production?
Oh, scaling up solid-state batteries is a breeze—if you enjoy complex, expensive puzzles! You face challenges like intricate fabrication, costly materials, and needing advanced facilities.
You also have to prevent moisture exposure, improve interface stability, and avoid lithium dendrites. Plus, higher costs, demanding thermal management, and the need for huge investments make going big a formidable task.
How Do Solid-State Batteries Perform in Extreme Temperature Conditions?
You might wonder how solid-state batteries handle extreme temperatures. At low temperatures, their ionic resistance rises, reducing power and capacity, which can limit your vehicle’s performance without thermal management.
At high temperatures, risks include electrolyte degradation and mechanical stress, threatening safety and lifespan. Advances in materials and design aim to expand their temperature range, helping you benefit from more reliable, safer, and efficient battery performance across diverse conditions.
When Will Commercial Solid-State Batteries Become Widely Available?
You might think solid-state batteries are just around the corner, ready to revolutionize your ride, but reality’s a bit slower. Despite early hopes, large-scale commercial availability isn’t until between 2027 and 2030.
Companies like Nissan and Honda plan around 2028-2029, but manufacturing hurdles and qualification processes mean you’ll still be waiting a little longer.
Conclusion
As you explore solid-state batteries, remember they’re the dawn of a new era in energy storage—bright with promise yet still needing to overcome hurdles. With advances in materials, manufacturing, and industry investment, you’ll find this technology shaping the future of electric vehicles and beyond. Keep an eye on emerging innovations; they’re the seeds that will grow tomorrow’s power solutions, transforming the landscape like a phoenix rising from the ashes.