Antiviral medications work by targeting specific stages of a virus’s life cycle, stopping it from infecting cells or replicating. They usually block virus attachment, prevent entry into host cells, interfere with genome replication, or stop protein assembly. The chemical structures of these drugs help them bind precisely to viral enzymes or proteins, making them effective. If you stay curious, you’ll discover how these medicines can be designed to overcome resistance and improve treatments.
Key Takeaways
- Antiviral drugs interfere with specific stages of the viral life cycle, such as attachment, entry, uncoating, and replication.
- Many antivirals mimic natural viral substrates, binding to viral enzymes to inhibit their activity through competitive or allosteric interactions.
- Structural modifications of antiviral molecules enhance their ability to selectively target viral proteins while improving stability and membrane permeability.
- Combining drugs that target multiple viral stages reduces resistance development and improves overall treatment effectiveness.
- Some antivirals boost the host immune response, activating antiviral genes and immune cells to help clear infections.
Blocking Virus Attachment and Entry Into Host Cells
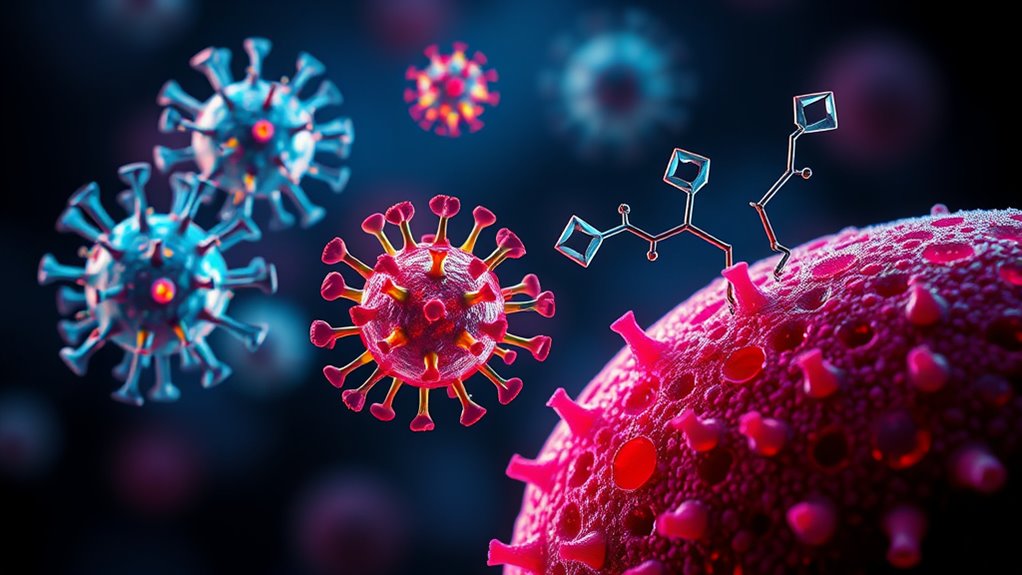
Blocking virus attachment and entry into host cells is a critical step in preventing viral infections. You need to understand that viruses attach to specific receptor molecules on your cells to begin infection. Antiviral agents can block this process by targeting either the viral proteins or your cell receptors involved in binding. Entry inhibitors work by preventing virus-receptor interactions, stopping the virus from entering your cells altogether. Some drugs bind to host receptors, inactivating them, while others mimic receptors to trap the virus before it reaches your cells. Developing these agents can be complex and costly, given the variability in viral attachment mechanisms. Understanding the specific receptors involved, such as CD4, CCR5, or CXCR4, is essential for designing effective entry inhibitors. Additionally, advances in molecular biology have facilitated the identification of novel targets for antiviral drugs, making the development of entry inhibitors more feasible. Nevertheless, targeting attachment offers a promising way to halt infection early, preventing the virus from establishing itself in your body.
Disrupting Viral Genome Replication Processes
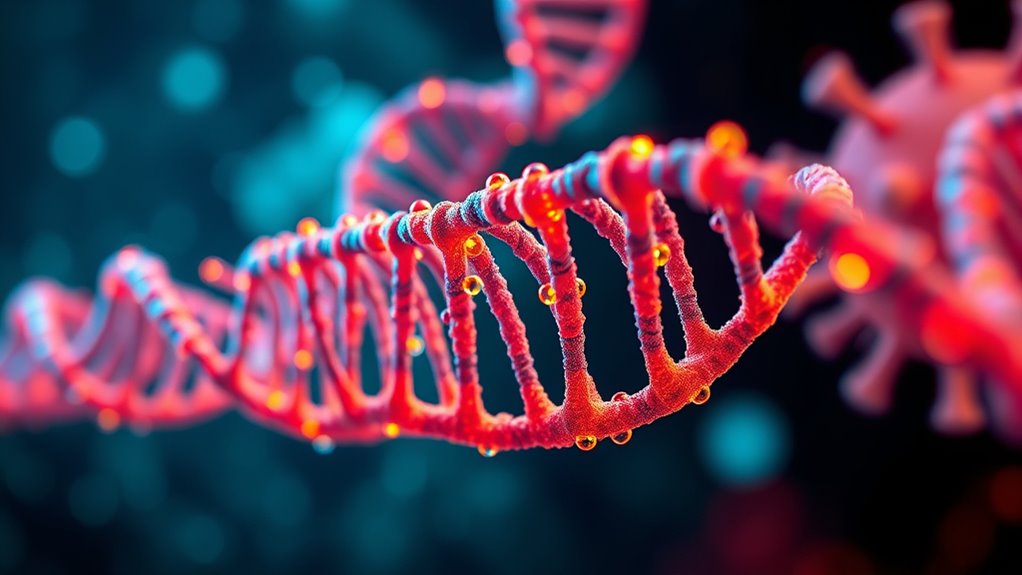
Disrupting viral genome replication is a key strategy in antiviral therapy, aiming to halt the production of new viral particles. You do this by targeting viral enzymes like RNA polymerase, preventing the synthesis of viral RNA or DNA. Viral genome packaging is a critical step in the viral life cycle, involving the folding of viral RNA segments into specific structures and their assembly into new viral particles. Nucleoside and nucleotide analogs, such as remdesivir, mimic natural building blocks, causing chain termination or errors during replication. These drugs often need to be converted into their triphosphate forms to effectively inhibit viral synthesis. Some antivirals introduce mutations into the viral genome, producing non-infectious particles. Broad-spectrum drugs like ribavirin can target multiple viruses by disrupting their replication processes. Understanding the viral replication mechanisms helps scientists develop more effective antiviral drugs with fewer side effects.
Inhibiting Viral Protein Maturation and Assembly
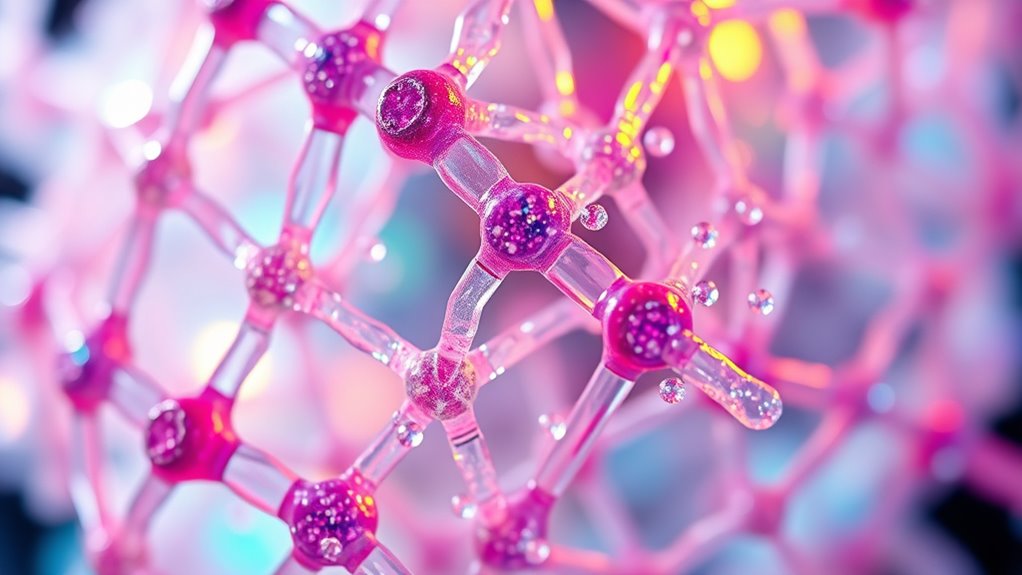
Inhibiting viral protein maturation and assembly is a crucial strategy in antiviral therapy, targeting the final steps of the viral life cycle to prevent infectious particle formation. You can use drugs that bind to viral structural proteins, such as pleconaril, which attaches to the capsid of rhinovirus and poliovirus, blocking RNA release. Other antivirals stabilize protein-protein interactions or induce conformational changes, disrupting proper assembly. Maturation inhibitors like bevirimat target HIV’s Gag protein, stopping virus maturation. In hepatitis B, core protein allosteric modulators cause abnormal capsid formation. Peptide inhibitors also show promise by interfering with assembly processes, offering broad-spectrum potential. These approaches aim to prevent the formation of functional, infectious viruses, halting their spread effectively. This method can also reduce the likelihood of resistance development by targeting multiple steps in the assembly process. Additionally, understanding the chemistry of protein interactions is essential for designing effective antiviral compounds.
Boosting the Host’s Immune Defense Mechanisms
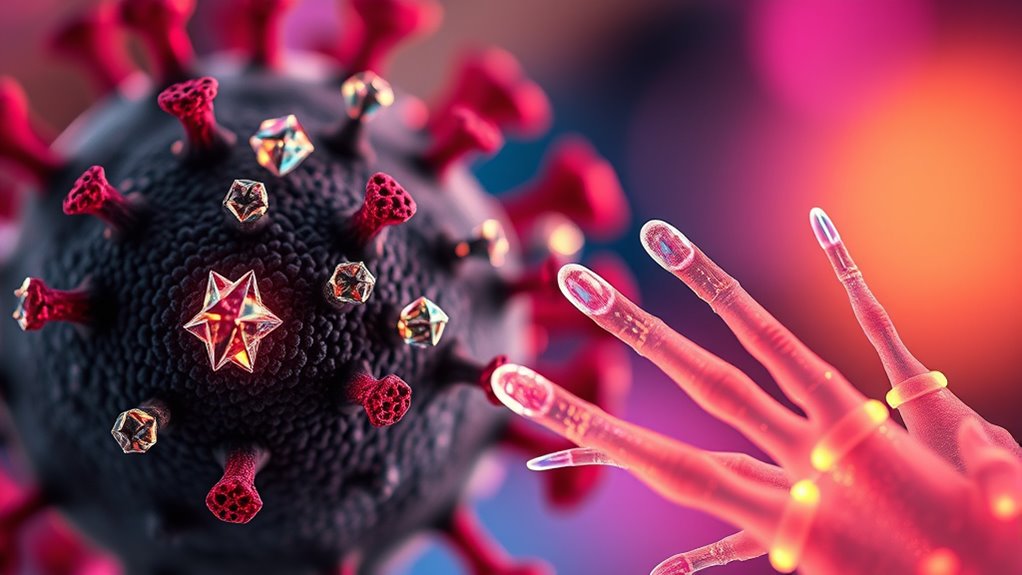
Boosting the host’s immune defense mechanisms offers a powerful approach to fighting viral infections by enhancing your body’s natural ability to detect and eliminate pathogens. You can stimulate type-I interferon production, activating antiviral genes in neighboring cells and triggering JAK-STAT signaling to amplify immune responses. This boosts cellular resistance by blocking viral replication and promoting antiviral states through interferon-stimulated genes like PKR. You also enhance immune cell activity: recruiting natural killer cells to destroy infected cells, activating macrophages for phagocytosis, and stimulating neutrophil traps to neutralize viruses. Additionally, promoting dendritic cell maturation improves T-cell responses, while increasing complement activity helps opsonize viruses. Enhancing vaccine responses can also be achieved through immune boosting strategies, which can provide additional protection against future infections. Furthermore, the modulation of cytokines plays a crucial role in immune regulation, ensuring a balanced response that effectively targets viruses without excessive inflammation.
Preventing the Release and Spread of Virus Particles
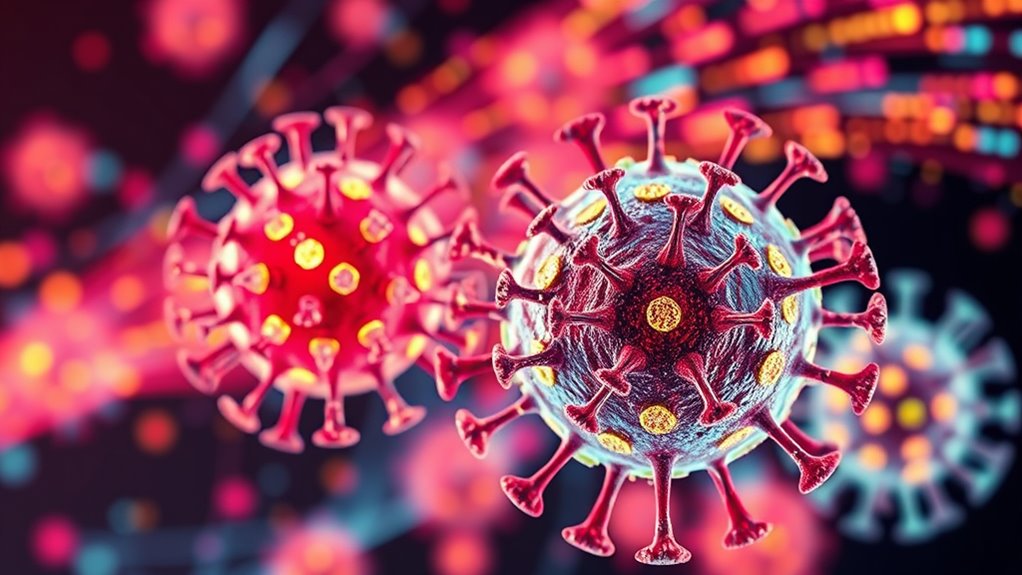
While strengthening your immune defenses helps your body fight off viruses, another key strategy focuses on stopping the virus from spreading once it’s inside your cells. Certain antivirals block the release of new viral particles from infected cells, reducing infection spread. Antibiotic resistance, however, is not a concern with antivirals, but improper use can still lead to reduced effectiveness. For example, neuraminidase inhibitors like oseltamivir prevent influenza viruses from detaching, causing particles to stay stuck at the cell surface. This limits the number of viruses available to infect neighboring cells, lowering your viral load and decreasing symptoms. By trapping viral particles, these drugs also reduce transmission risk to others. Since they target late-stage processes, they work alongside entry or replication inhibitors to provide a thorough approach. However, viruses can develop resistance, so monitoring and combination therapies are essential for maintaining effectiveness.
Overcoming Challenges and Resistance in Antiviral Therapy
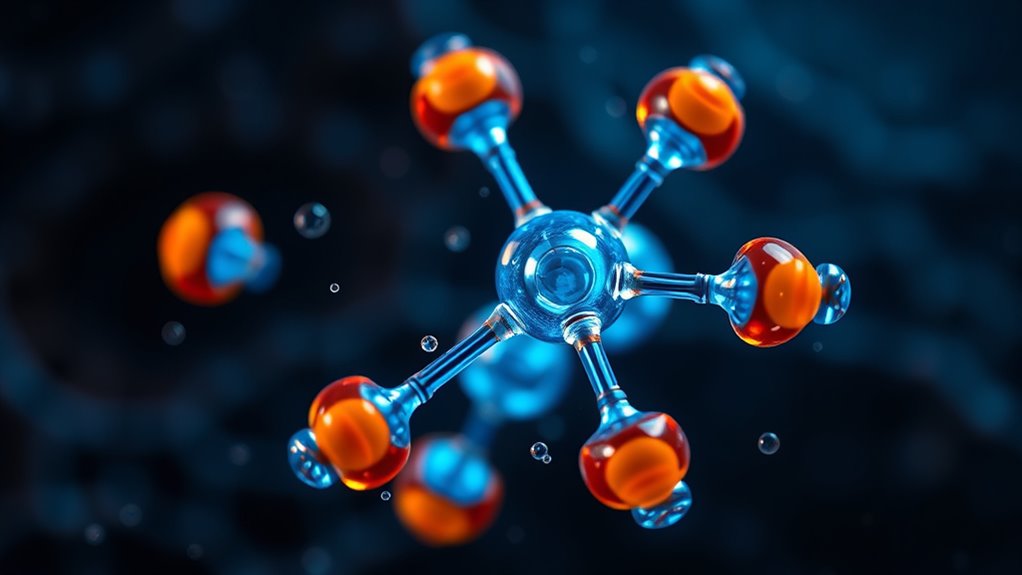
Overcoming resistance in antiviral therapy is essential for maintaining treatment effectiveness as viruses mutate and adapt. Resistance often results from mutations in viral genes that change drug targets or replication pathways. Prolonged drug use and ongoing viral replication, especially in immunosuppressed patients, increase resistance risks. Less potent regimens allow viruses to continue replicating, raising the chance of resistance development. To combat this, combination therapies target multiple viral processes, and more potent antivirals improve suppression. Understanding resistance mechanisms guides drug design, while monitoring viral strains helps adjust treatments. Innovative delivery systems also enhance drug efficacy. Additionally, viral mutation patterns provide insights into potential resistance development, guiding more effective treatment strategies. Here’s a quick overview:
Strategy | Purpose | Outcome |
---|---|---|
Combination therapies | Delay resistance development | Prolonged drug effectiveness |
Higher potency antivirals | Reduce viral replication | Minimize resistance emergence |
Resistance monitoring | Detect resistance patterns early | Adjust treatment promptly |
Advanced delivery systems | Improve drug bioavailability | Increase treatment success |
Developing new antivirals that target multiple stages of the viral lifecycle is also crucial for staying ahead of resistance.
Targeting Specific Stages of the Viral Life Cycle
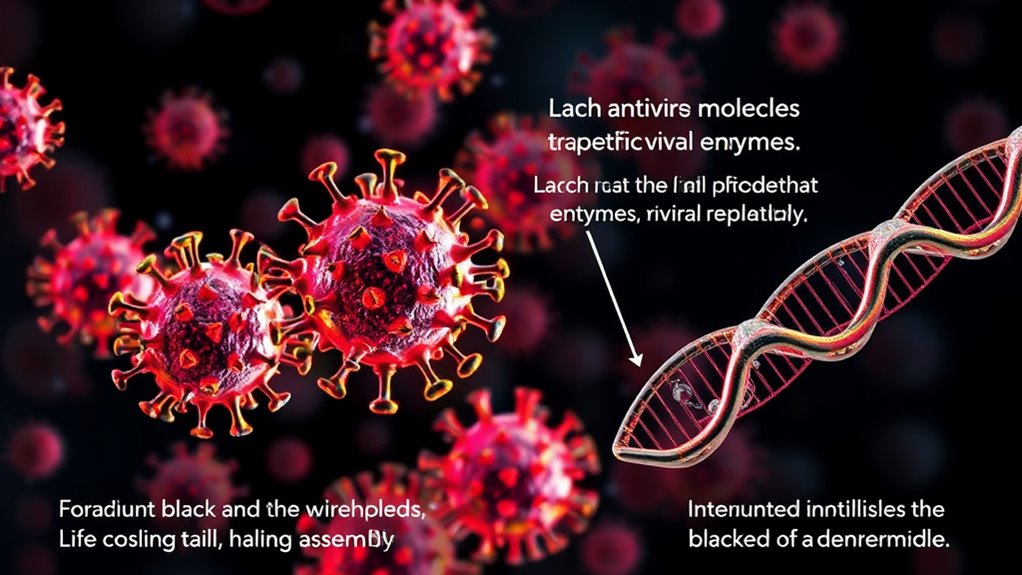
Targeting specific stages of the viral life cycle allows antiviral therapies to intervene at critical points, preventing the virus from replicating and spreading within the host.
By blocking viral attachment, these drugs stop the virus from binding to host cell receptors, halting infection early. Entry inhibitors prevent the fusion of viral and host membranes, while uncoating inhibitors stabilize the viral capsid, stopping genetic material release.
Inhibiting genome replication involves targeting viral enzymes like reverse transcriptase or polymerases, reducing viral production. Some antivirals interfere with viral protein synthesis, preventing the formation of mature particles. Others block assembly, budding, or release, such as neuraminidase inhibitors that prevent influenza viruses from exiting cells.
This multi-stage approach effectively limits viral proliferation and disease progression. Incorporating knowledge of viral structures and the antiviral mechanism helps in designing more effective treatments.
The Role of Chemical Structures in Antiviral Effectiveness
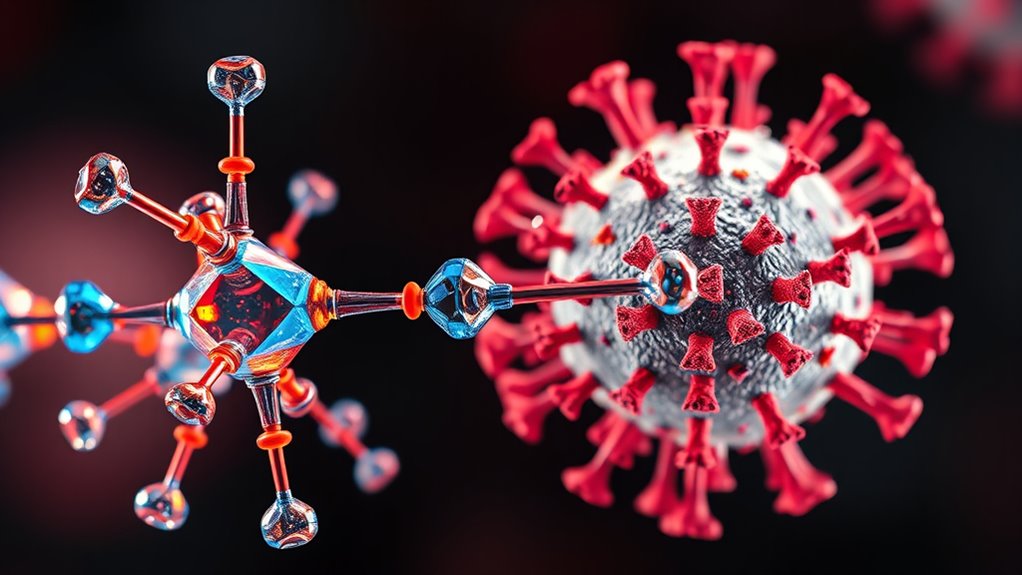
The chemical structures of antiviral drugs directly influence their ability to interact with and inhibit viral proteins or enzymes essential for replication. You’ll notice that many antivirals mimic natural substrates or cofactors, enabling them to competitively block viral enzymes. Storing essential oils tips highlight the importance of proper storage to maintain potency, which is analogous to how the structural integrity of drugs affects their effectiveness. Functional groups like hydroxyl or amine facilitate specific interactions—hydrogen bonds, hydrophobic contacts, or ionic bonds—with viral targets. The drug’s conformational flexibility or rigidity determines how well it fits into active or allosteric sites of viral proteins. By optimizing these structures, chemists improve drug potency and selectivity, which reduces side effects. Structural modifications, such as adding chemical groups or adjusting stereochemistry, enhance binding affinity, stability, and membrane permeability. This precise tailoring of chemical structures ensures antivirals are more effective, selective, and safer in fighting viral infections.
Frequently Asked Questions
How Do Antiviral Drugs Differentiate Between Viral and Host Cell Components?
You might wonder how antiviral drugs target viruses without harming your cells. They achieve this by focusing on viral-specific enzymes and processes, like unique polymerases, proteases, or entry mechanisms.
These drugs block these viral components directly, or interfere with steps like fusion or genome integration, which your cells don’t do.
This selective targeting minimizes damage to your healthy cells while effectively stopping the virus in its tracks.
Can Antiviral Medications Be Effective Against Multiple Virus Families?
You might wonder if antiviral medications can work against multiple virus families. The answer is yes, especially with broad-spectrum antivirals designed to target conserved viral structures like RNA polymerases.
These drugs interfere with viral replication across different viruses by mimicking nucleic acids or inhibiting key enzymes. While promising, they face challenges like resistance and limited targets, so ongoing research aims to improve their effectiveness and develop more versatile treatments.
What Are the Latest Advancements in Designing Broad-Spectrum Antivirals?
Imagine holding the key to stopping countless viruses—today’s advancements make that possible. You now see innovative strategies targeting viral fusion and envelope features, rather than specific viruses.
AI-driven design helps create broad-spectrum antivirals like Bioxytran’s Prolectin-M, effective against multiple viruses. These drugs aim to block virus entry, reducing resistance.
With public-private collaborations and faster development, you’re at the forefront of a new era in pandemic-ready treatments.
How Do Mutations in Viruses Impact the Effectiveness of Antiviral Treatments?
Mutations in viruses can profoundly affect how well antiviral treatments work. When mutations alter key enzymes or surface proteins, they can make drugs less effective or completely ineffective.
You might see resistance develop, especially if the virus mutates in areas targeted by the medication. Rapid mutations, like in RNA viruses, increase this risk, so staying aware of new mutations is essential to adjust treatments and maintain their effectiveness.
Are There Natural Compounds With Antiviral Properties Similar to Pharmaceutical Drugs?
You might be surprised to learn that natural compounds do have antiviral properties similar to pharmaceutical drugs. Plants and marine organisms produce bioactive substances like alkaloids, flavonoids, and terpenoids that can inhibit viruses by blocking attachment, replication, or enzymes.
These natural agents often act synergistically, boosting your immune response while directly fighting the virus. They’re safer, more affordable, and provide unique structures that can inspire new antiviral medications.
Conclusion
Understanding how antiviral medications work helps you appreciate their role in fighting infections. Did you know that over 50 antiviral drugs are approved worldwide, each targeting different stages of the viral life cycle? By blocking entry, replication, or release, these drugs effectively reduce illness severity. Staying informed about their mechanisms empowers you to make better health decisions and supports ongoing research to overcome resistance and improve treatments.